The Welding of Stainless Steels
Reprinted from Euro Inox with permission.
Author: Pierre-Jean Cunat, Joinville-le-Pont, France
Acknowledgement: The paragraphs marked (*) in the text are extracted from “Working with Stainless Steels”, Paris (SIRPE) 1998
Title Photograph: ESAB AB, Göteborg (S)
Contents
1 General information on stainless steels
2 Stainless steel welding processes
3 Weldability of stainless steels
4 Selecting shielding gases for welding of stainless steels
5 Selecting welding consumables for welding of stainless steels
6 Joint preparation in arc welding
7 Finishing treatments for welds
8 Safe practices
9 Glossary: terms and definitions
1 General Information on Stainless Steels
1.1 Austenitic Stainless Steels: Fe-Cr-Ni (Mo)
These alloys are the most popular grades of stainless steels because of their excellent formability, corrosion resistance, and weldability. All are non-magnetic in the annealed condition.
Range of compositions: C ≤ 0.10% - 16% ≤ Cr ≤ 28% - 3.5% ≤ Ni ≤ 32% - (Mo ≤ 7%).
1.2 Ferritic Stainless Steels: Fe-Cr-(Mo)
Ferritic stainless steels have a low carbon content, with chromium (and molybdenum) as major alloying elements.
They are non-hardenable by heat treatment and are always magnetic.
Range of compositions: C ≤ 0.08% - 10.5% ≤ Cr ≤ 30% - (Mo ≤ 4.5%).
1.3 Austenitic - Ferritic Duplex Stainless Steels: Fe-Cr-Ni (Mo)-N
The microstructure of duplex stainless steels consists of a mixture of austenite and ferrite. They exhibit characteristics of both phases with higher strength and ductility. Nitrogen is added to provide strength and also aids in weldability. They are magnetic and non-hardenable by heat treatment.
Range of compositions: C ≤ 0.03% - 21% ≤ Cr ≤ 26% - 3.5% ≤ Ni ≤ 8% - (Mo ≤ 4.5%) - N ≤ 0.35%.
1.4 Martensitic Stainless Steels: Fe-Cr-(Mo-Ni-V)
These alloys can be heat treated to a wide range of useful hardness levels.
The martensic structure obtained is magnetic.
Range of compositions: C ≤ 1.2% - 11.5% ≤ Cr ≤ 17% - (Mo ≤ 1.8% - Ni ≤ 6% - V ≤ 0.2%).
2 Stainless Steel Welding Processes
2.1 Electric Arc Processes
2.1.1 Processes with a Refractory Metal Electrode
2.1.1.1 Gas Tungsten Arc Welding: GTAW (*)
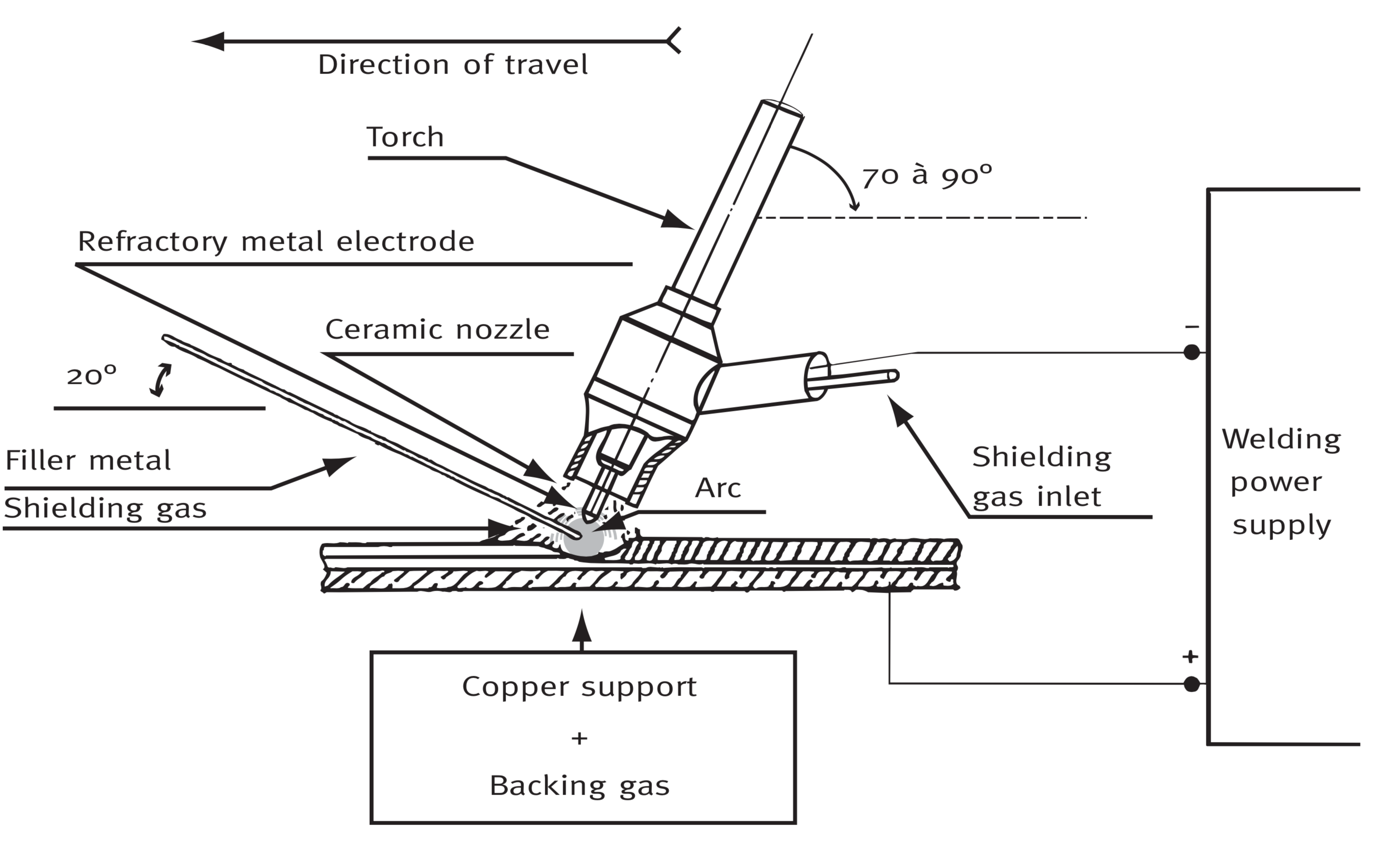
The GTAW process, also known as the TIG (Tungsten Inert Gas) or WIG (Wolfram Inert Gas) process, is illustrated in the above figure. The energy necessary for melting the metal is supplied by an electric arc struck and maintained between a tungsten or tungsten alloy electrode and the workpiece, under an inert or slightly reducing atmosphere. Stainless steels are always welded in the DCEN (Direct Current Electrode Negative) or DCSP (Direct Current Straight Polarity) mode. In these conditions, it is the workpiece that is struck by the electrons, enhancing penetration, while the electrode, which is generally made from thoriated tungsten (2% ThO2 ), undergoes very little wear. If a filler metal is employed, it is in the form of either bare rods or coiled wire for automatic welding. The inert gas flow which protects the arc zone from the ambient air, enables a very stable arc to be maintained. Depending on the base material, shielding gases consist mainly of mixtures of argon (Ar), helium (He) and hydrogen (H2) (see section 4 ‘Selection of shielding gases for welding stainless steel’).
The main advantages of this process when used on stainless steels can be summarised as follows:
- a concentrated heat source, leading to a narrow fusion zone;
- a very stable arc and calm welding pool of small size. Spatter is absent and because no flux is required in the process, oxidation residues are eliminated so that any final cleaning operation is very much simplified;
- an excellent metallurgical quality, with a precise control of penetration and weld shape in all positions;
- sound and pore-free welds
- very low electrode wear
- easy apprenticeship
The common workpiece thickness range is 0.5 mm to 3.5 / 4.0 mm.
2.1.1.2 Plasma Arc Welding: PAW (*)
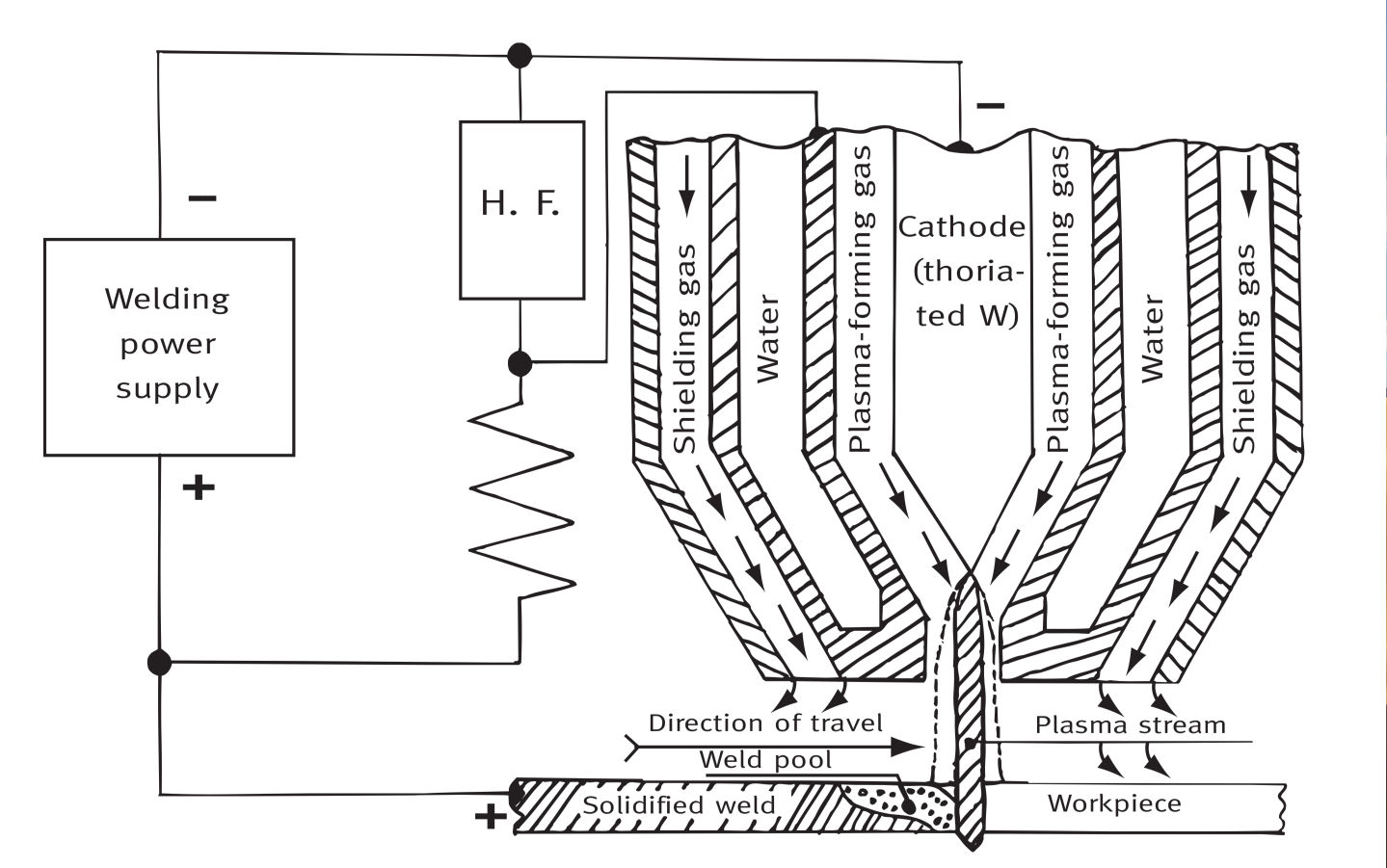
Plasma welding is similar to Gas Tungsten Arc Welding (GTAW). The significant difference is that the arc plasma is constricted by a nozzle to produce a high-energy plasma stream in which temperatures between 10,000 and 20,000°C are attained.
Welding processes generally employ a ‘transferred arc’ configuration, where the constricted arc is formed between the electrode and the workpiece, whereas other applications more often use a ‘non-transferred’ constricted arc.
Since the plasma jet is extremely narrow, it cannot provide adequate protection for the weld pool, so that it is necessary to add a larger diameter annular stream of shielding gas.
The gases used both for this purpose and for forming the plasma are similar to those employed in GTAW, namely pure argon (Ar), Ar – hydrogen (H2) up to 20%, Ar-helium (He) – H2. The hydrogen-containing mixtures are recommended for welding austenitic stainless steels, but like in the case of GTAW, are be to proscribed for ferritic, martensitic and duplex grades. For the latter materials, it is recommended to add nitrogen to maintain the appropriate pro- portions of austenite and ferrite in the weld (see section 4 ‘Selecting shielding gases for welding stainless steels’).
In manual plasma welding, where the torch is hand-held, the so-called ‘micro-plasma’ and ‘mini-plasma’ processes are employed for currents between 0.1 and 15 amperes and the ‘non-emergent jet’ technique for currents between about 15 and 100 amperes.
In automatic welding, where the torch is mounted on a carriage, the so-called ‘key-hole’ process is employed. By increasing the welding current (above 100 amperes) and plasma gas flow, a very powerful plasma beam is created which can achieve full penetration in the workpiece. During welding, the hole progressively cuts through the metal with the weld pool flowing in behind to form the weld.
The major advantage of the PAW process over GTAW is the remarkable stability of the arc leading to:
- a ‘rigid’ arc which enables better control of power input;
- greater tolerance to variations in nozzle- workpiece distance, without significant modification to the weld morphology;
- a narrow heat-affected zone (HAZ) and generally faster welding speeds;
- greater tolerance to faulty preparation, particularly in the case of keyhole welding.
The common workpiece thickness range is:
- 0.1 mm to 1.0 mm for micro-plasma and mini-plasma processes
- 1.0 mm to 3.5 mm for the non-emergent jet technique
- 3.5 mm to 10.0 mm for the keyhole process (in a single pass).
2.1.2 Processes with a Fusible Electrode
2.1.2.1 Gas Metal Arc Welding: GMAW (*)
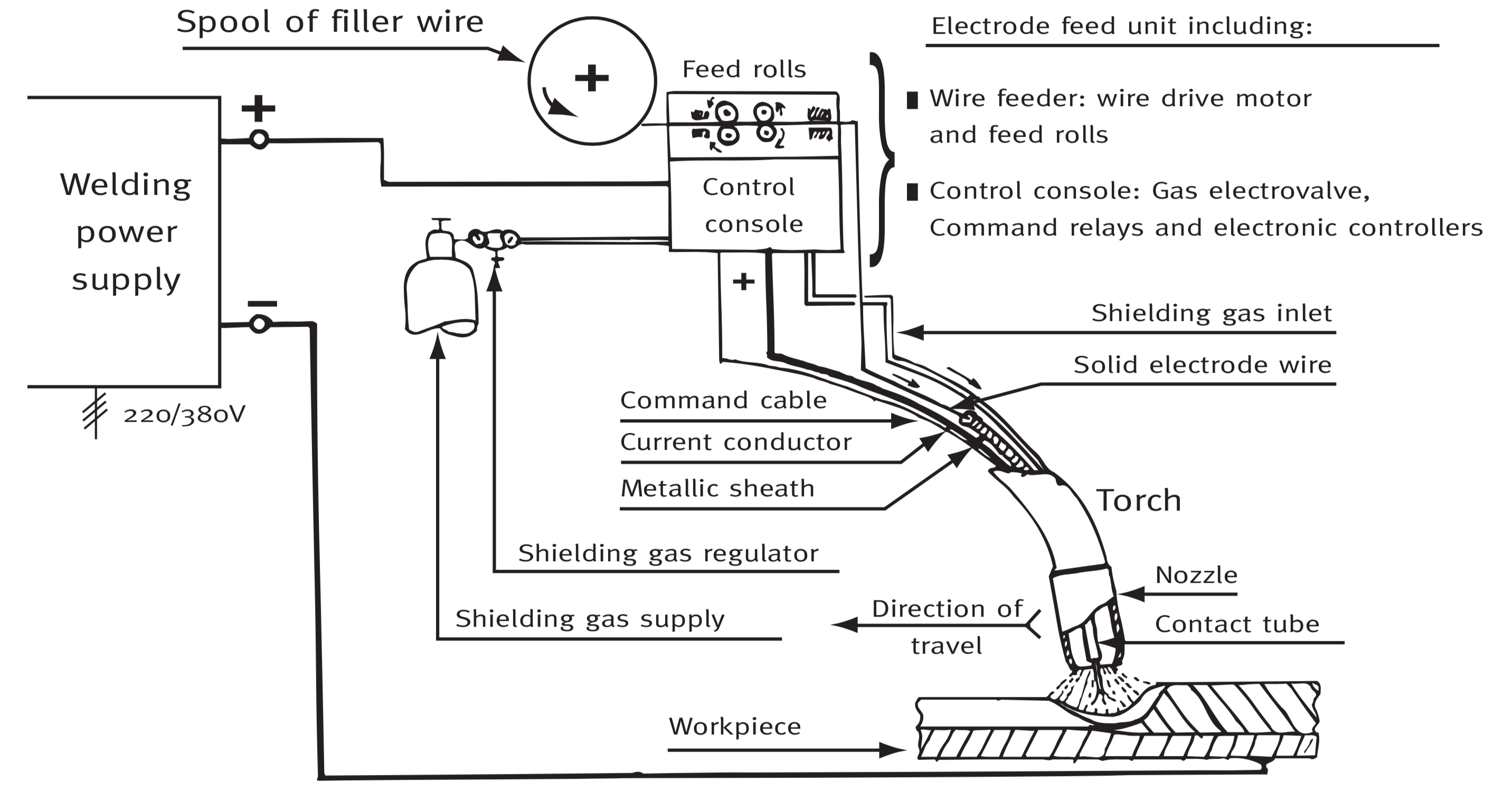
In the GMAW process, also known as to MIG (Metal Inert Gas) process, the welding heat is produced by an arc struck between a continuously fed metal wire electrode and the workpiece.
Contrary to the GTAW and PAW processes, the electrode is consumable, an arc being struck between the fusible filler wire and the workpiece under a shielding gas.
The essential characteristics of this process are:
- the use of very high current densities in the electrode wire (>90A/mm2), about 10 times higher than in the covered electrode (SMAW) process;
- rapid melting of the electrode wire (melting rate of about 8 m/min) due to the high temperature of the arc, making it necessary to use an automatic wire feed system, supplied by 12 kg. spools;
- stainless steels are always welded in the DCEP (Direct Current Electrode Positive) or DCRP (Direct Current Reverse Polarity) mode, the positive pole of the generator being connected to the electrode;
- the welding torch is generally held manually (so-called ‘semi-automatic’ process), but for high welding powers it is fixed to a carriage (‘automatic’ process).
The mechanism of metal transfer in the arc is an important process parameter, three principle modes being distinguished:
The short-circuiting or dip transfer mode, in which the metal melts to form large droplets whose diameter is often greater than that of the electrode wire. As the droplet forms at the end of the electrode, it makes contact with the weld pool and creates a short circuit, with a sudden increase in current. The surface tension causes a pinching effect which separates the droplet from the electrode. The frequency of this phenomenon is of the order of 20 to 100 Hz, corresponding to cycle times between 0.01 and 0.05 seconds. The globular transfer or gravity transfer mode. As in the previous case, melting occurs in the form of large droplets, which break away when their mass is sufficient to overcome surface tension forces and due to the greater arc length, fall freely before coming into contact with the weld pool. The spray transfer mode involves cur- rent densities above a certain transition level, of the order of 200 A/mm2. The electrode melts to give a stream of fine droplets. As the current density increases further, the electrode tip becomes conical and the stream of even finer droplets is released axially.
GMAW requires a shielding gas to prevent oxidation in the welding arc (see section 4 ‘Selecting shielding gases for welding stainless steels’). Argon with 2% oxygen (O2) gives a stable arc and is suitable for most applications. Argon with 3% carbon dioxide (CO2) gives about the same result. The welding speed and penetration can sometimes be increased when helium (He) and hydrogen (H2) are added to the argon + O2 or argon + CO2 shielding gas. Gases higher in CO2 (MAG process) tend to produce significant carbon pickup by the weld pool together with chromium oxidation. It is for this reason that they are not recommended.
The bead size and extent of penetration, will vary according to the workpiece grade (ferritics, austenitics, etc.), the type of joint, the transfer mode and the skill of the welder. For single V joints and square butt joints welded in one run, the common workpiece thickness range is 1.0 mm to 5.0 mm.
Note: The GMAW process is frequently referred to as MIG welding. Confusion often arises between MIG and MAG welding processes. In fact, in the MIG process, the oxidising nature of the shielding gas (see section ‘Selecting gases for welding stainless steels’) is negligible, whereas it is deliberately enhanced in the MAG process. However, in the GMAW / MIG process, a low percentage of oxygen (O2) or carbon dioxide (CO2) is often needed in the shielding gas (argon) to improve both arc stability and wetting by the molten metal. Typical levels are 2% O2 or 3% CO2. Higher levels of O2 or CO2 give excessive oxidation of chromium (Cr), manganese (Mn) and silicon (Si) and excessive pick- up of carbon (C) in the weld pool. For example, the carbon content (% C) in the weld metal, which is 0.025% for 2% CO2 containing shielding gas, could reach 0.04% for 4% CO2.
2.1.2.2 Flux Cored Arc Welding: FCAW (*)
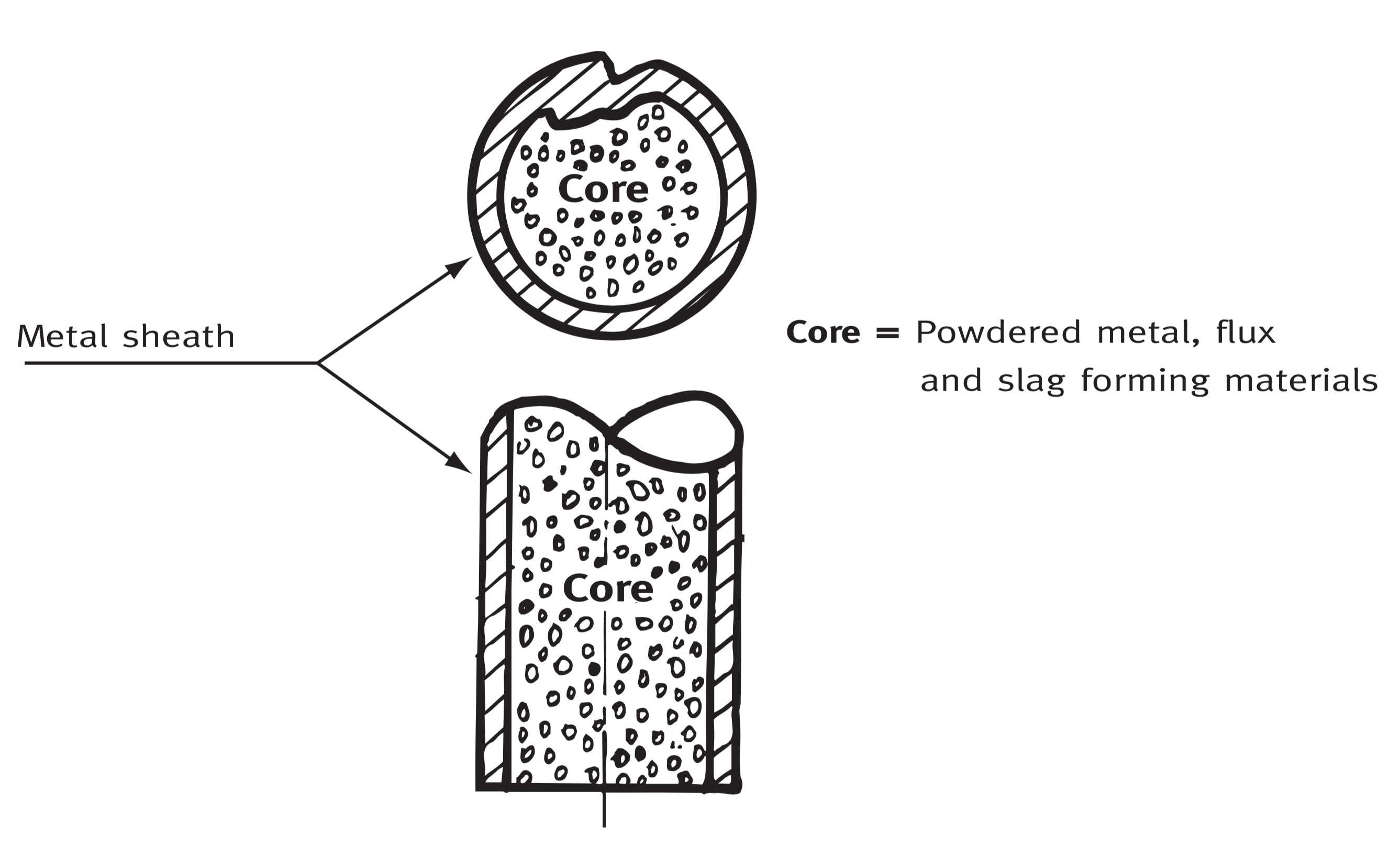
A variant of the GMAW process is the FCAW (Flux Cored Arc Welding) process, in which the electrode wire is composed of a stain- less steel sheath filled with a solid flux, whose role is similar to that of the electrode covering in the manual SMAW process. The core provides deoxidizers and slag forming materials and may provide shielding gases in the case of self-shielded FCAW electrodes.
The FCAW technique combines the advantages of the SMAW method with the high productivity of an automatic or semi-automatic process due to the possibility of continuously feeding the cored wire. Compared to a conventional solid electrode, the flux provides a slag cover and enhances productivity.
Thus, for a current of about 200 amperes, the deposition rate is about 100 g / min. for a solid 1.6 mm diameter wire containing 20% Cr and 10% Ni, compared to about 170 g / min for a flux-cored wire of the same diameter. This large difference is due to the fact that in the flux-cored wire, only the metal sheath conducts electricity, since the core, composed of a mixture of mineral and metal powders, possibly bound in an alkali silicate, has a high electrical resistivity.
Both FCAW and GMAW have similar bead sizes. For single V joints and square butt joints welded in one run, the common workpiece thickness range is 1.0 mm to 5.0 mm.
2.1.2.3 Shielded Metal Arc Welding (covered Electrode): SMAW (*)
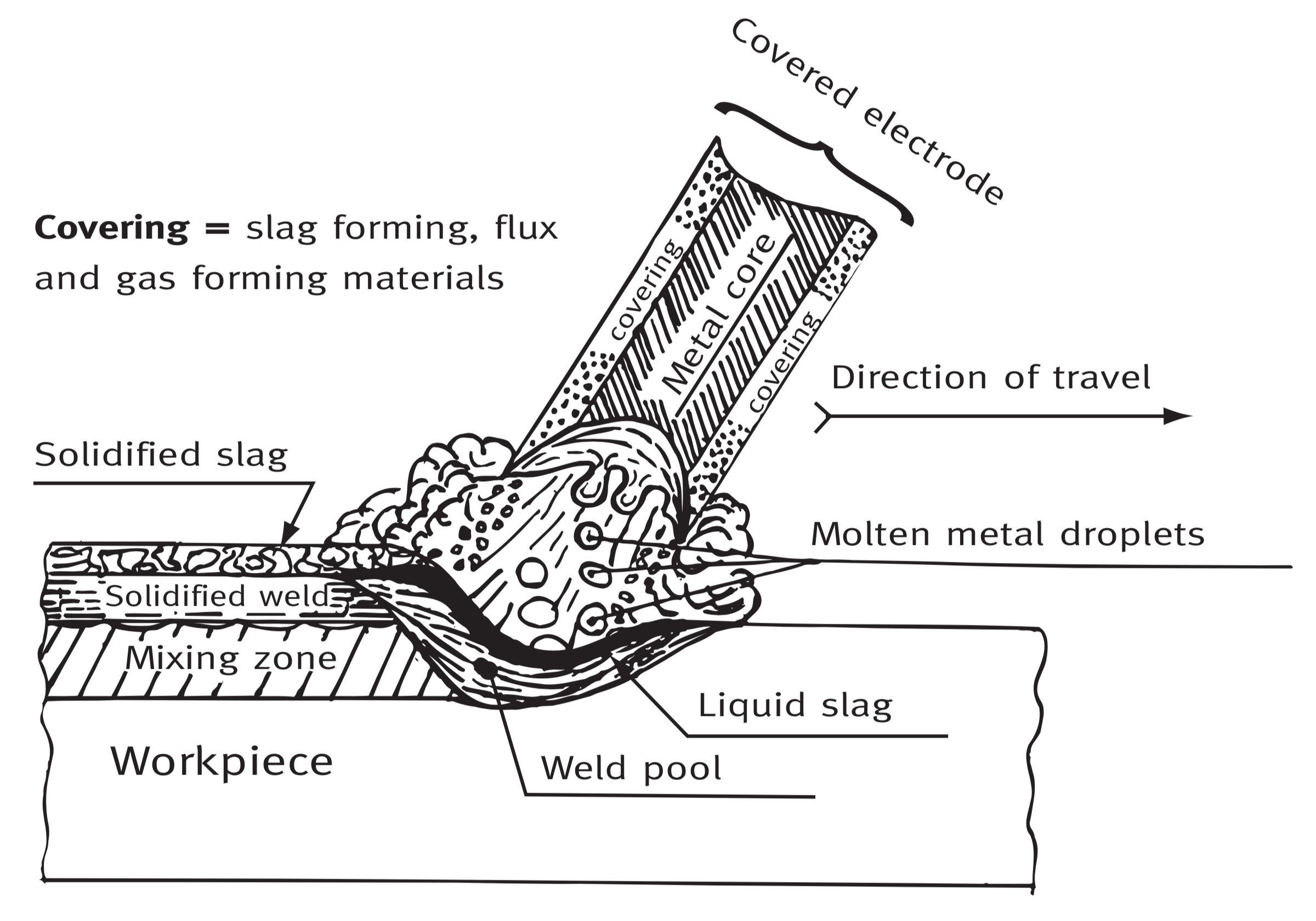
Although the SMAW process, also known as the MMA (Manual Metal Arc) process is very old, since the first applications were reported by Kjelberg in 1907, it remains widely employed due to its great flexibility and simplicity of use.
The electrode consists of a metal core covered with a layer of flux. The core is usually a solid stainless steel wire rod. The covering, which plays on essential role in the process, is extruded onto the core, and gives each electrode its specific ‘personality’. It serves three main functions: electrical, physical and metallurgical. The electrical function is related to initiation and stabilisation of the arc, while the physical action concerns the viscosity and surface tension of the slag, which control the transfer of metal droplets, the effective shielding of the weld pool and its wettability. The metallurgical role involves chemical exchanges between the weld pool and the slag, i.e. refining of the weld metal.
The covering contains a certain amount of calcium carbonate (CaCO3) which dissociates in the arc at about 900°C, to form CaO and CO2, the latter ensuring protection of the arc zone. The following section gives a short description of the most frequently used covered-electrodes:
- Rutile (titania) electrodes: Slag formation is the main shielding mechanism in rutile based electrodes. Rutile electrodes are easy to handle, ensure low spatter and produce welds with smooth surfaces. The slag formed during the welding operation is easy to remove;
- Basic (lime) electrodes: Limestone is the main constituent of basic covered electrodes due to its favourable arc-stabilizing and metallurgical characteristics. It also evolves carbon dioxide which provides a gas shield. However, a major disadvantage of limestone is its high melting point. This is counteracted by additions of fluorspar (CaF2) which helps to lower the slag melting point. Basic coverings will absorb moisture if they are left in the open air for any length of time, and special care should be taken to keep the electrode dry.
The typical drying time is one hour at a temperature of approximately 150°C to 250°C.
Rutile covered electrodes can be employed in both the AC and DC modes whereas basic (lime covered) electrodes are used essentially in the DCEP mode.
The common workpiece thickness range is:
1.0 mm to 2.5 mm for single run processes
3.0 mm to 10.0 mm for a multipass technique
2.1.2.4 Submerged Arc Welding: SAW (*)
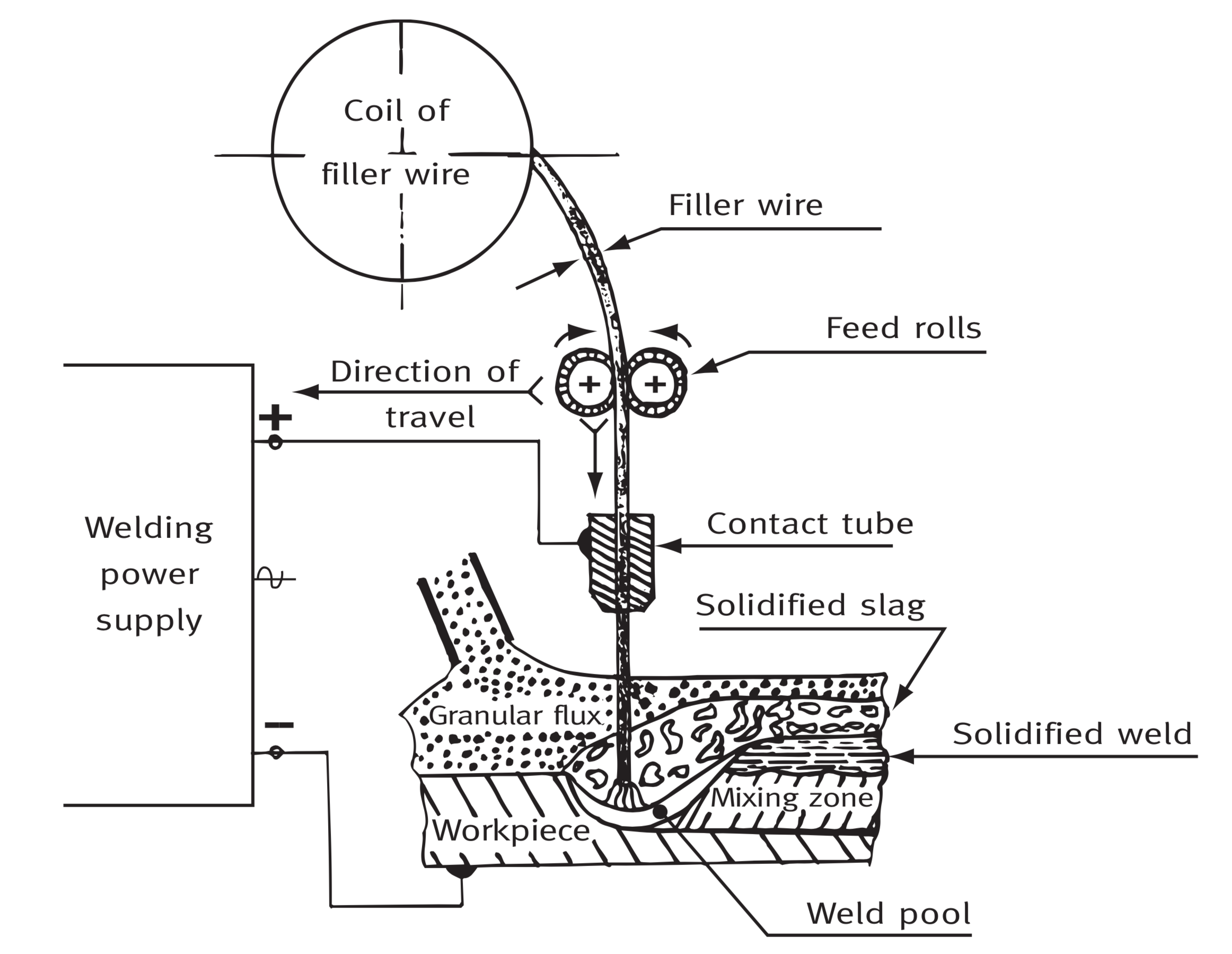
In the SAW process the welding heat is generated by the passage of a heavy electric current between one or several continuous wires and the workpiece under a powdered flux which forms a protective molten slag covering.
The process may be either fully or semi-automatic, although in the case of stainless steels most work is done with fully automatic equipment.
In the automatic process, the welding current can be very high, up to 2,000 amperes per wire, leading to a large power input and consequently a heavy dilution of the base metal by the filler material.
The process is suitable for butt and fillet welding in the flat position and horizontal – vertical fillet welding. The power supply is generally of the DCEP reverse polarity type, and more rarely AC, when several wires are employed simultaneously in order to avoid arc blow phenomena. For both DC and AC generators, the electrode wire pay out speed must be equal to the melting rate in order to obtain a perfectly stable arc. This is achieved by the use of feed rolls commanded by a motor reducing gear system with servo-controlled speed. For welding stainless steels, a ‘lime/fluoride’ type flux is most widely used, its typical composition being:
25% ≤ CaO + MgO ≤ 40%, SiO2 ≤ 15%, 20% ≤ CaF2 ≤ 35%.
Two forms exist, produced either by melting or bonding. Fused fluxes are produced by heating to temperatures of the order of 1,600 – 1,700°C, and are converted to powder form either by atomisation on leaving the melting furnace, or by crushing and screening the solidified bulk material. Bonded fluxes are produced from raw materials of appropriate grain size, bonded together with an alkali silicate binder. The mixture obtained is dried, then mechanically treated, to obtain the desired final particle size.
Only part of the flux is fused during welding and the unfused material is picked up, usually by a suction hose and returned to a hopper for further use. The fused flux solidifies behind the welding zone and on cooling contracts and can be readily detached.
For thicker material, welds are usually made in one or two passes, i.e. a single run on a manual backing weld, or one run from either side of the plate, but a multipass technique may also be employed. In thinner material, welds can be made in one run with the aid of a grooved backing strip.
Since the SAW process is used mainly for thick austenitic stainless steel sheet, particular care must be taken to avoid the formation of sigma phase due to the use of high welding energies. This is especially the case for 25% Cr – 20% Ni alloys, but also for 18% Cr – 9% Ni grades with high ferrite contents. In multipass welding, where the temperature range 650 – 900°C is crossed several times, the risk of sigma phase formation is increased. Subsequent solution annealing at 1,050°C is then highly recommended.
In the as-delivered condition, the fluxes are perfectly dry. In order to prevent moisture pick-up, it is recommended to store the flux at a temperature about 10°C higher than that of the workshop, in an atmosphere whose relative humidity does not exceed 50%.
If moisture pick-up is feared or suspected, it is useful to bake the powder at 300°C for at least two hours.
The SAW process is generally used for joining heavy workpieces in the thickness range 10 – 80 mm, after the root run has been completed using another welding process. The bottom bead may also be made with the aid of a grooved backing strip.
2.1.2.5 Stud Welding: SW
Stud welding is a method of attaching a metal stud to a workpiece generally in the form of a sheet or a plate. There are two distinct stud welding methods: arc welding (ARC) and capacitor discharge (CD).
- Arc Stud Welding (ARC) involves the same basic principles and metallurgical aspects as any other arc welding procedure. The stud is placed in contact with the workpiece using a hand tool called the stud gun, and an arc is struck which melts the stud base and an area of the workpiece. Before welding, a ceramic ferrule is placed in position over the end of the stud to shield the arc and to confine the weld metal.
- The stud is then forced into the weld pool and held in place until the molten metal solidifies and forms a homogeneous joint. The cycle is completed in less than a second, producing a full strength joint. The expandable ferrule is broken away to expose a smooth and complete fillet at the stud base.
- Capacitor discharge (CD) stud welding involves the same basic principles and metallurgical aspects as any other arc welding procedure. When the weld gun is activated, a special precision weld tip initiates a controlled electric arc from the welder capacitor bank which melts the end of the stud and a portion of the workpiece. The stud is held in place as the molten metal solidifies, instantly creating a high quality fusion weld. Since the entire weld cycle is completed in several milliseconds, welds can be made to thin sheet without pronounced distortion, burn-through or discoloration and with small diameter fasteners (9 mm and less). CD welding also permits stud welding of dissimilar metallic alloys.
ARC or CD Process?
The arc process is generally used for stud diameters of 6 mm and above and when welding to thicker base materials or in structural applications.
The CD technique is generally used for stud diameters up to 9 mm and is employed primarily when welding to thin sheet metal.
Stainless Steel Studs
Most stainless steels can be stud welded. With the exception of free machining grades, austenitic stainless steels studs are most commonly used for stud welding.
Stainless steel studs are currently welded to stainless steels and can also be welded to mild steel. In this case, it is essential that the carbon content of the base metal does not exceed 0.20%.
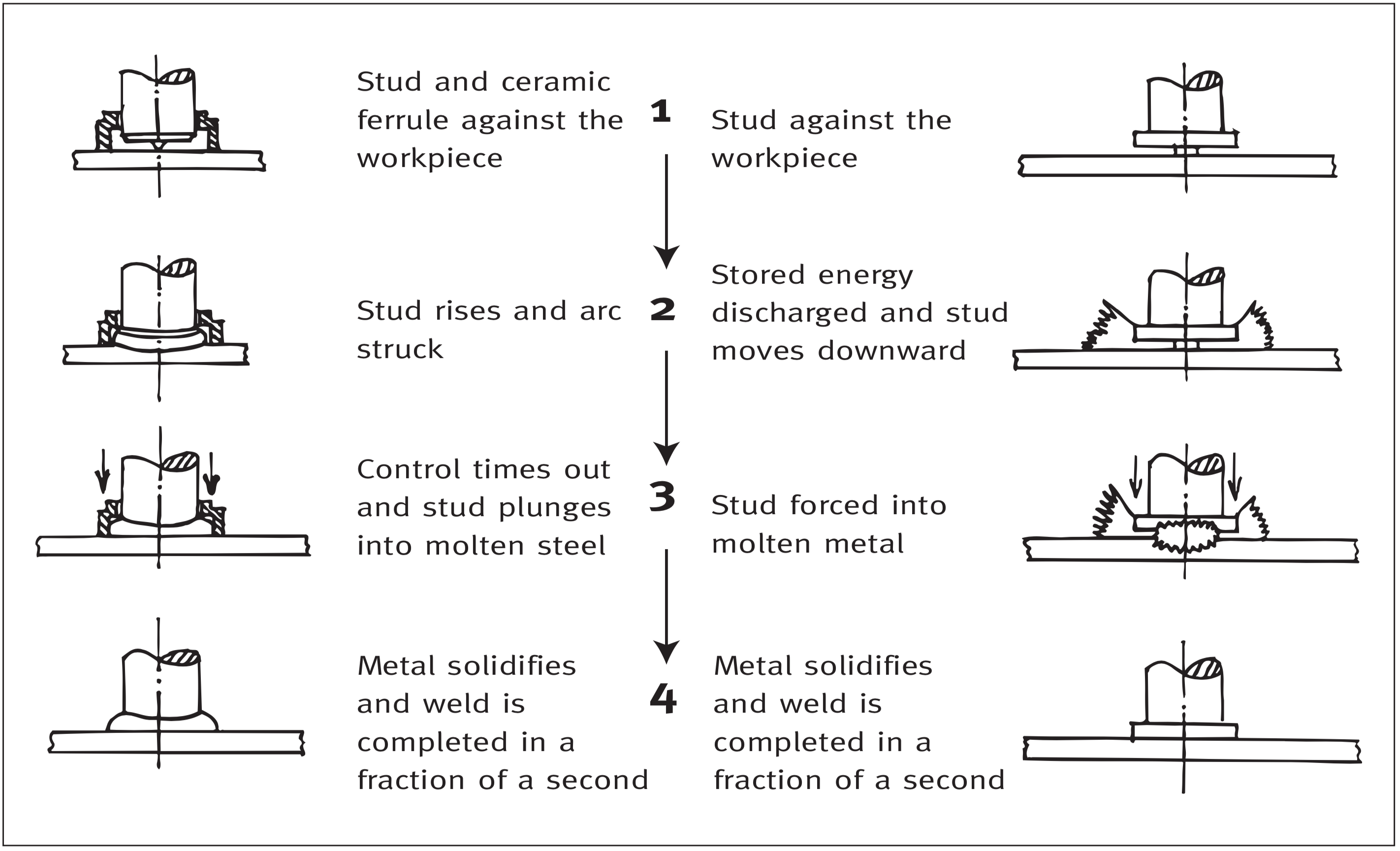
2.2 Resistance and Induction Processes
2.2.1 Resistance Spot Welding: RSW (*)
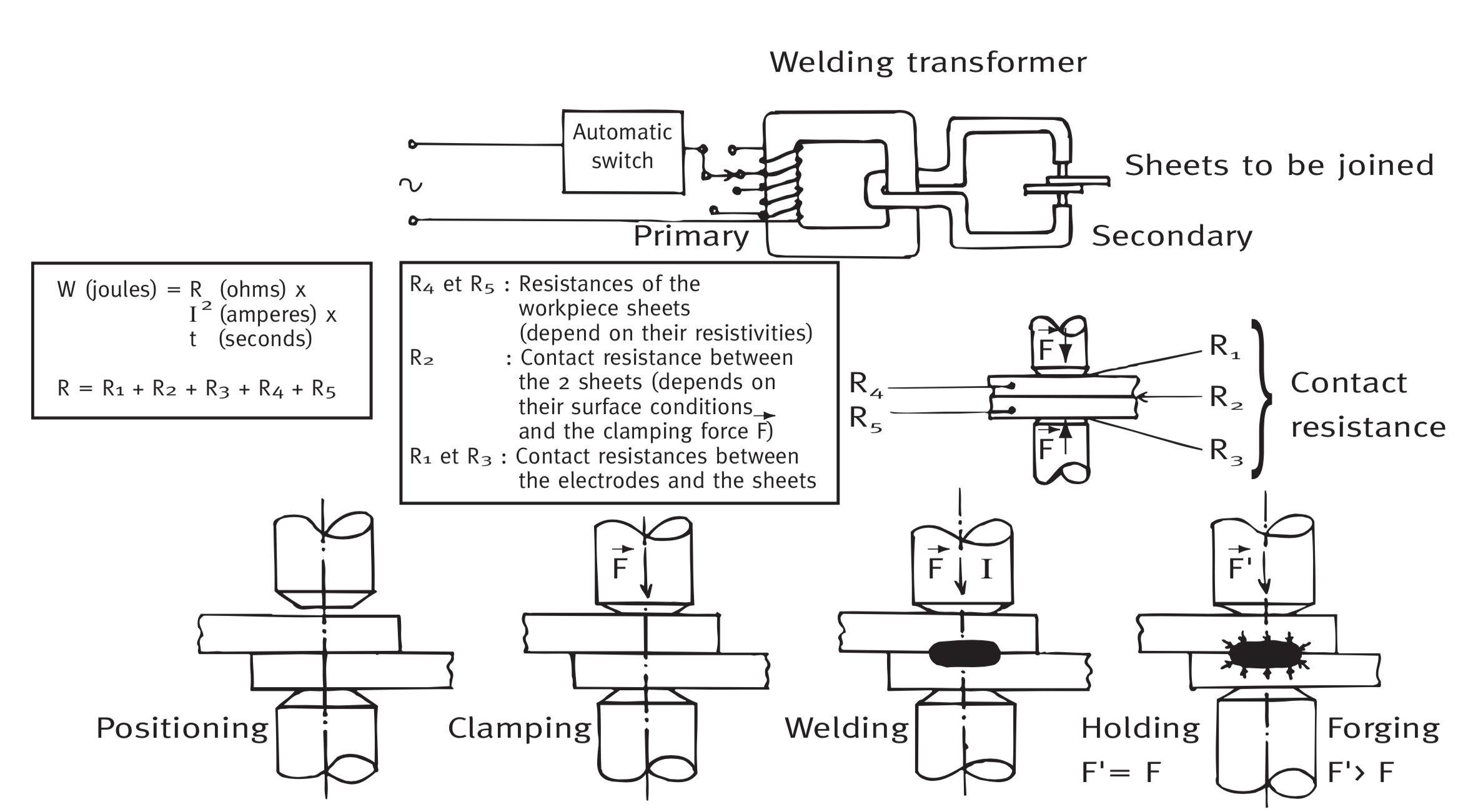
This process is still extensively used and is particularly suited to the welding of thin stainless steel sheets. Melting is induced by resistance heating due to the passage of an electric current through the workpiece materials at the joint.
Five different stages are generally distinguished in the spot welding process, namely:
- Positioning of the sheets to be joined
- Lowering of the upper electrode and application of the clamping force
- Welding with a low voltage alternating current, producing a heat energy W (joules) = R (ohms) x I2 (amperes) x t (seconds)
- Holding of the clamping force or application of an additional forging force and finally
- Raising of the upper electrode before proceeding to the next cycle.
With regard to the electrode materials, in the case of stainless steels, the best combination of low resistivity and high mechanical strength is obtained with copper – cobalt – beryllium alloys. The electrode tips are generally in the form of a truncated cone with an open angle of 120°. Formation of the weld nugget depends on the welding current and its duration and on the clamping force applied by the electrodes.
The welding parameters recommended for 18% Cr – 9% Ni austenitic stainless steel and stabilised 17% Cr ferritic grades are indicated in the following table.
Sheet Thickness (mm) | Electrode Tip Diameter (mm) | Electrode Clamping Force (daN) | Welding Current (A) | Welding Time (no. of Periods) |
---|---|---|---|---|
18% Cr – 9% Ni | Austenitic | Grades | ||
0.5 | 3.0 | 170 | 3,500 | 3 |
0.8 | 4.5 | 300 | 6,000 | 4 |
2.0 | 6.0 | 650 | 11,000 | 8 |
Stabilised | 17% Cr Ferritic | Grades | ||
0.5 | 3.0 | 150 | 4,000 | 3 |
0.8 | 4.5 | 250 | 7,550 | 4 |
The parameters given in the above table must be optimised to allow for the surface condition (pickled, glazed, bright annealed, polished), which has a strong influence on the interface resistance, which in turn plays a decisive role in nugget formation.
Contrary to other fusion welding processes, in resistance spot welding, the melt pool cannot be controlled visually. The only defects perceptible to the eye are an excessive electrode indentation and surface spatter. However, a simple albeit destructive inspection method is the so-called ‘peel test’, which gives a rapid indication of the quality of the spot weld. In this test, one of the welded sheets is peeled off the other so that ‘buttons’ of metal tend to be pulled from one or other of the sheets.
2.2.2 Resistance Seam Welding: RSEW (*)
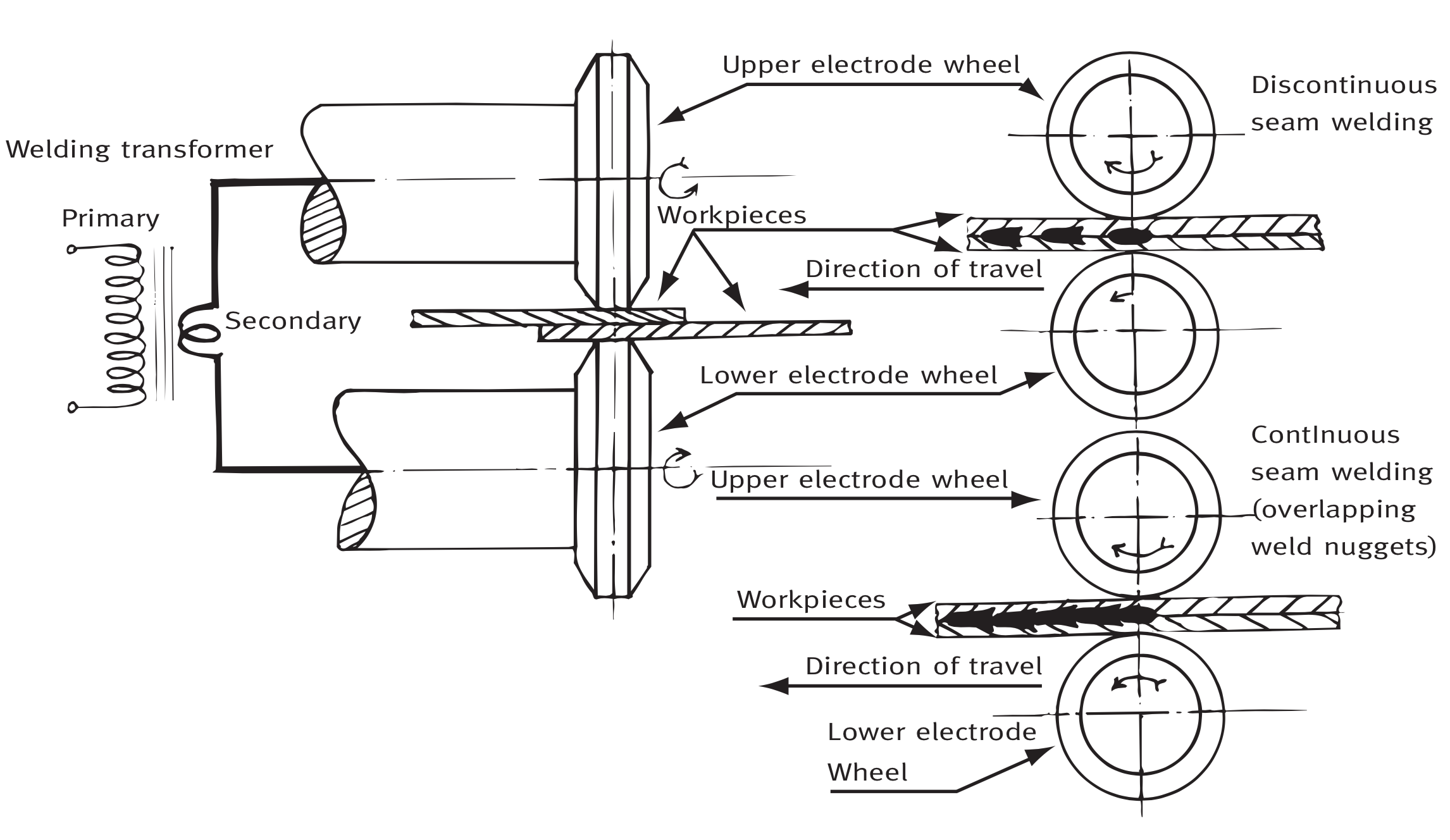
The principle of resistance seam welding is similar to that of spot welding, except that the process is continuous. The major difference is in the type of electrodes, which are two copper-alloy wheels equipped with an appropriate drive system. The wheel edges usually have either a double chamfer or a convex profile. Compared to spot welding, where the principal process parameters are the welding current, the heating time and the hold time, additional factors to be considered in seam welding are the use of a modulated or pulsed current and the welding speed.
The welding parameters recommended for Fe-Cr-Ni austenitic grades are indicated in the following table.
Sheet Thickness (mm) | Wheel Thickness (mm) | Clamping Force (daN) | Welding Time (periods) | Off Time (periods) | Welding Current (Amp) | Welding Speed (cm/min) |
---|---|---|---|---|---|---|
0.5 | 3.0 | 320 | 3 | 2 | 7,900 | 140 |
0.8 | 4.5 | 460 | 3 | 3 | 10,600 | 120 |
1.5 | 6.5 | 80 | 3 | 4 | 15,000 | 100 |
2.0 | 8.0 | 1,200 | 4 | 5 | 16,700 | 95 |
3.0 | 9.5 | 1,500 | 5 | 7 | 17,000 | 95 |
In both spot and seam welding, the major advantages of electrical resistance heating are:
- the limited modification of the microstructure in the heat affected zones,
- the virtual absence of surface oxidation if the sheets are correctly cooled (by streaming cold water)
- the very small distortion of the sheets after welding.
2.2.3 Projection Welding: PW (*)
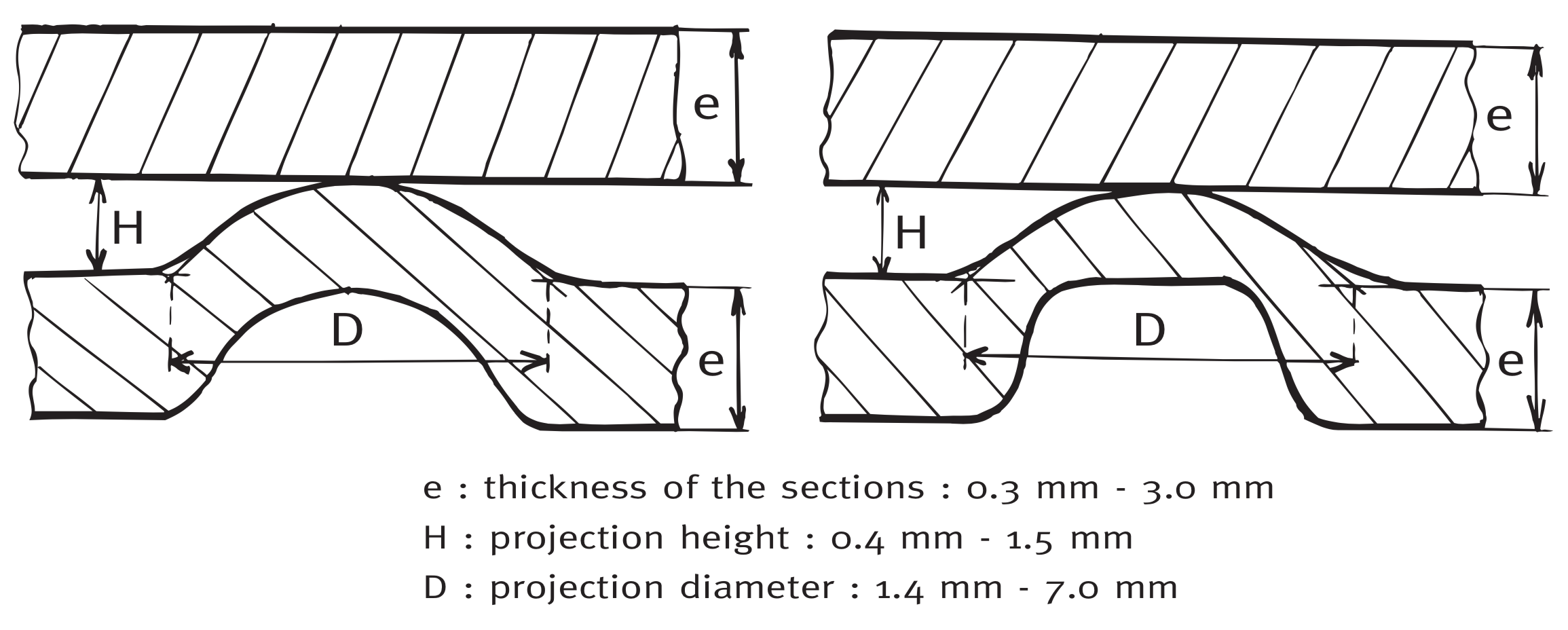
In this process, small prepared projections on one of the two workpiece surfaces are melted and collapse when current is supplied through flat copper-alloy electrodes. The projections are formed by embossing (sheet metal parts) or machining (solid metal parts) usually on the thicker or higher electrical conductivity workpiece of the joint. The projections are shaped and positioned to concentrate the current and a large number of spot welds can be made simultaneously. Lower currents and pressures are used than for sport welding in order to avoid collapse of the projections before the opposite workpiece surface has melted. The welding time is about the same for single or multiple projections of the same design.
Projection welding is especially useful for producing several weld spots simultaneously between two workpieces.
Various designs of weld fasteners are available for annular projection welding applications; e.g. shoulder-studs, bolts, pins, nuts and pads.
2.2.4 Electroslag Welding: ESW
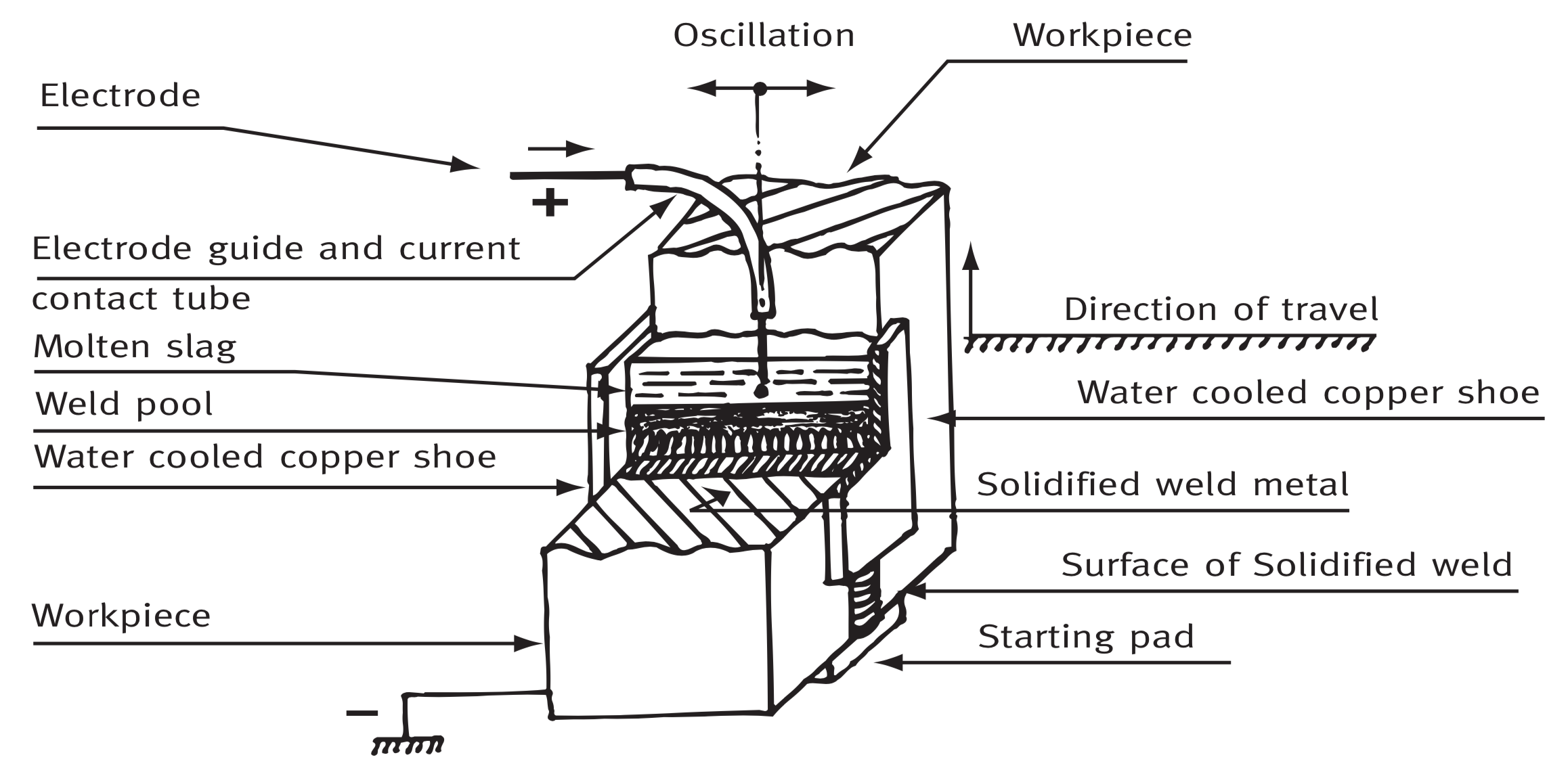
Principle of the electroslag welding process
The electroslag welding process was developed at the E.O. Paton Welding Institute (Ukraine) in the early 1950's.
Electroslag welding is a single pass process used to produce butt joints in the vertical position. Joints thicker than 15mm (with no upper thickness limit) can be welded in one pass, and a simple square-edged joint preparation is required. The process is similar to a vertical casting operation since the molten weld metal is contained by the two workpiece plates and by a pair of cooled copper shoes.
Except during startup of the electroslag operation, there is no arc. The continuously fed electrodes are melted off by electrical resistance heating as they pass through a conductive molten slag layer (slag bath).
The slag bath also melts the adjacent workpiece plate edges and shields the molten metal from the atmosphere. The temperature of the bath is of the order of 1,900°C.
To start the electroslag process, a layer of flux is placed in the bottom of the joint and an arc is struck between the electrodes and the starting block or starting pad to provide a molten slag bath.
As welding proceeds, the copper shoes and the wire feed unit are moved up the joint at speeds of the order of 30 mm/min. The metal deposition rate is about 350 g/min. The electrode wire compositions normally match those of the base metal. The most popular electrode sizes are 1.6 mm, 2.4 mm and 3.2 mm in diameter.
The metallurgical structure of electroslag joints is unlike other fusion welds. The slow cooling and solidification can lead to a coarse grain structure. It is for this reason that the process is recommended only for austenitic grades.
2.2.5 Flash Welding: FW (*)
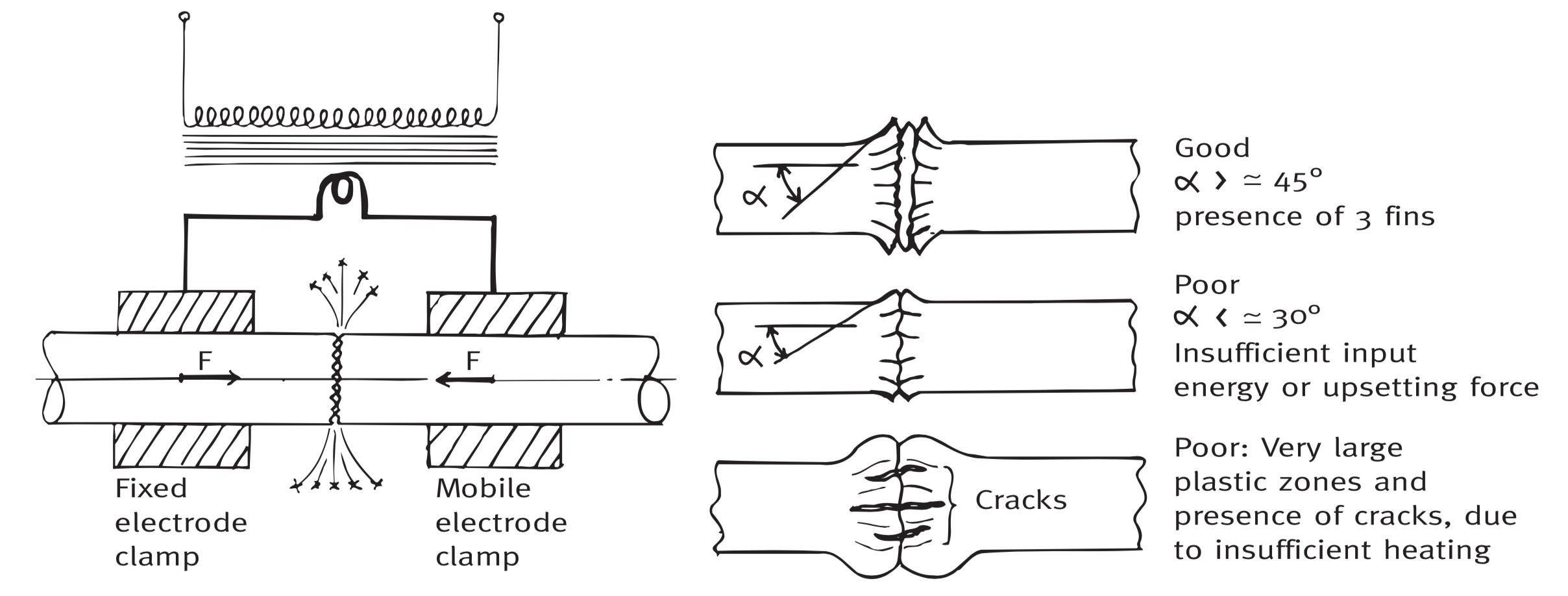
This technique is used essentially for long products, e.g. rods, bars, tubes and shaped sections. Although apparently similar to upset welding, flash butt welding is in fact quite different. Indeed, during upset welding, it has been observed that when the abutting surfaces are not in perfect contact, the current passes only in a few small areas, leading to intense local heating and rapid melting, creating arcs which violently expulse the molten metal out of the joint due to the associated magnetic fields (the flashing phenomenon).
The important process parameters are the welding current and the voltage, which must be sufficient to cause flashing, the instantaneous flashing speed, which must be proportional to the metal consumption and compensated by the travel of the mobile clamps, the duration of flashing and the final upset forging stage. The roughness of the initial faying surfaces must be such that the contact points are sufficiently numerous and well distributed to produce uniform flashing across the whole of the joint area.
After upset forging, the joint profile should show the characteristic three-finned profile indicative of a successful welding operation. The recommended welding parameters, as a function of section area, are given for austenitic grades in the following table.
Thickness (mm) | Section Area (mm2) | Initial Die Opening (mm) | Final Die Opening (mm) | Material Loss (Flashing & Forging) (mm) | Flash Time (sec) |
---|---|---|---|---|---|
2.0 | 40 | 13 | 5 | 8 | 2.2 |
5.0 | 570 | 25 | 7 | 18 | 6.0 |
10.0 | 1,700 | 40 | 15 | 25 | 17.0 |
Some typical applications are: wheel rims (for bicycles) produced from flash welded rings, rectangular frames (for windows and doors), etc.
2.2.6 High Frequency Induction Welding: HFIW (*)
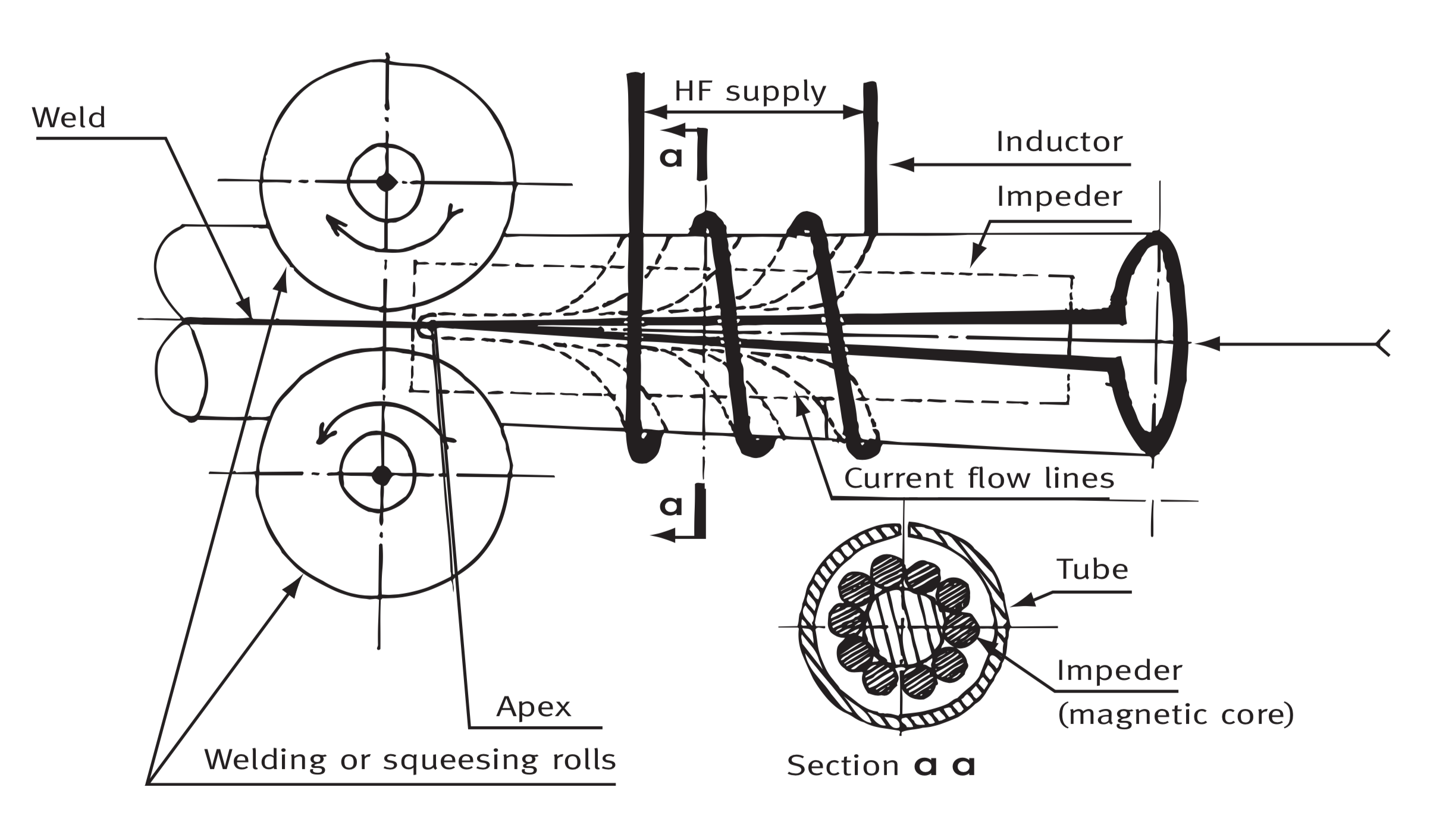
Principle of HF induction welding
High frequency induction welding is essentially used for making tubes from strip. The process is performed by a multiple roll forming system. On leaving the last rolling stand, the tube comprises a longitudinal slit which is closed by welding. The joint is formed by solid-solid contact, with intermediate melting, as the strip edges are brought together by a pair of horizontal rolls (squeezing rolls).
Due to the skin effect, the induced HF current (140 to 500 kHz) follows the path of minimum impedance, concentrating the heating at the edges.
In the case of ferritic stainless steels, this high productivity process avoids the grain coarsening phenomenon to which these grades are susceptible.
In this case, welding powers between 150 and 300 kW are employed depending on the tube diameter, the welding speed varying with the machine from 50 to 90 m/min.
2.3 Radiation Energy Processes (*)
2.3.1 Laser Beam Welding: LBW
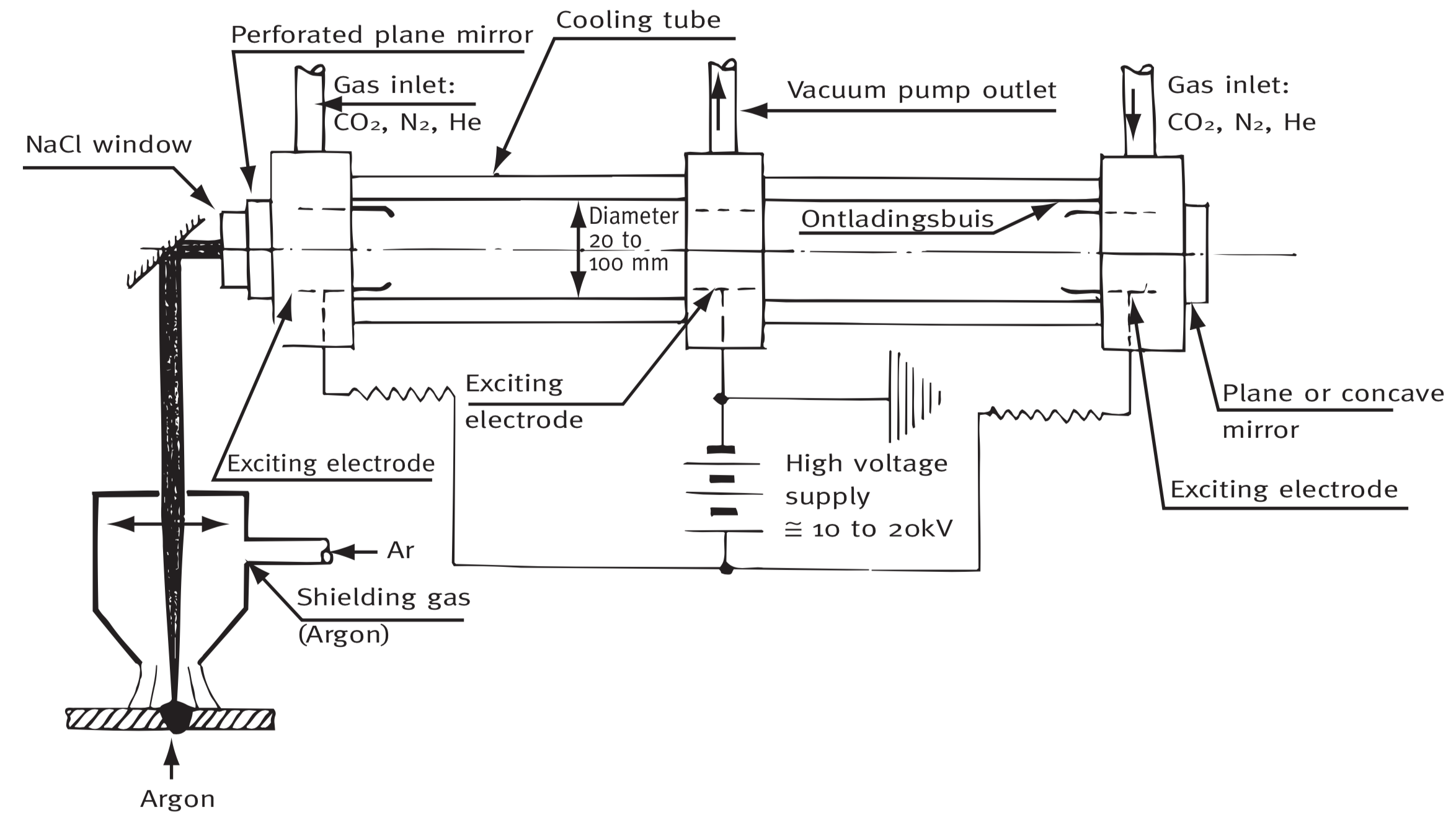
The laser effect (Light Amplification by Stimulated Emission of Radiation) was discovered in the optical wavelength range by Maiman in 1958. The possibility immediately appeared of using a laser beam as a small area contact–free high intensity power source for welding applications. The continuous power levels available are particularly high for carbon dioxide lasers, although it must be remembered that the effective welding power depends on the reflectivity of the workpiece material for a given incident wavelength.
The sources most widely used for welding purposes are CO2 gas lasers and solid state yttrium-aluminium garnet (YAG) lasers. YAG lasers are preferred for welding thin stainless steel sheets (< 1.5 mm) in the pulsed mode. So-called CO2 lasers are better adapted for the welding of thicker stainless steel sheets or strips (1.5 to 6.0 mm).
As in high frequency induction welding (HFIW), this process is widely used for the production of longitudinally welded tubes. With a power of about 6 kW, a 2 mm thick strip of stabilised 17% Cr ferritic stainless steel can be welded at a speed of about 7 m/min, and since the thermal cycle is very brief, grain coarsening in the heat affected zone is extremely limited.
2.3.2 Electron Beam Welding: EBW
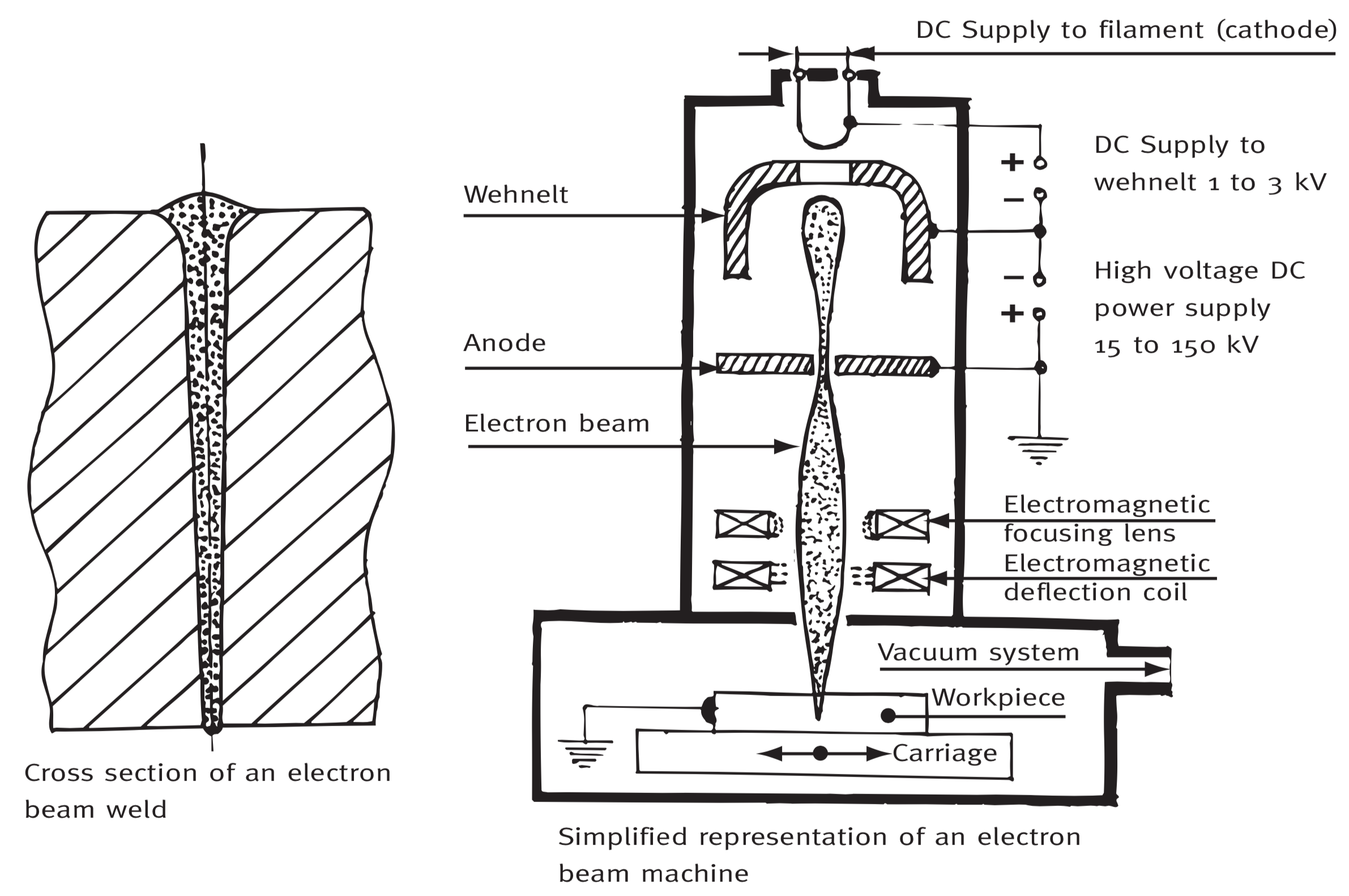
Electron beam welding uses energy from a high velocity focussed beam of electrons made to collide with the base material. With high beam energy, a hole can be melted through the material and penetrating welds can be formed at speeds of the order of 20 m / min.
EBW can produce deep and thin welds with narrow heat affected zones. The depth to width ratio is of the order of 20:1.
Welds are made in vacuum, which eliminates contamination of the weld pool by gases. The vacuum not only prevents weld contamination but produces a stable beam. The concentrated nature of the heat source makes the process very suitable for stainless steels. The available power can be readily controlled and the same welding machine can be applied to single pass welding of stainless steel in thicknesses from 0.5 mm to 40 mm.
3 Weldability of Stainless Steels
3.1 Austenitic Stainless Steels: Fe-Cr-Ni (Mo)-(N)
➤ Structures containing a few percent of ferrite (usual case)
- Insensitive to hot cracking
- Good resistance to intergranular corrosion for low carbon and stabilised grades
- Excellent toughness and ductility
- Embrittlement can occur after long exposures between 550 and 900°C due to decomposition of the ferrite to form sigma phase
➤ Fully austenitic structures (exceptional)
- Sensitive to hot cracking during solidification
- Good resistance to intergranular corrosion for low carbon and stabilised grades
- Excellent toughness and ductility
3.2 Ferritic Stainless Steels: Fe-Cr-(Mo-Ni-V)
➤ Semi ferritic grades: 0.04% C - 17% Cr
- Sensitive to embrittlement by grain coarsening above 1150°C
- Poor toughness and ductility
- Sensitive to intergranular corrosion
- Post-weld heat treatment at about 800°C restores the mechanical properties and intergranular corrosion resistance
➤ Ferritic grades: 0.02% C – 17-30% Cr – (Stabilised Ti, Nb)
- Sensitive to embrittlement by grain coarsening above 1,150°C
- Satisfactory ductility and improved toughness compared to semi-ferritic grades
- Generally insensitive to intergranular corrosion
3.3 Austenitic – Ferritic Duplex Stainless Steels: Fe-Cr-Ni (Mo)-N
- Insensitive to hot cracking
- Excellent toughness and good ductility in the range from –40°C to 275°C
- Sensitive to embrittlement by sigma phase when exposed between 500 and 900°C
3.4 Martensitic Stainless Steels: Fe-Cr-(Mo-Ni-V)
- Sensitive to cold cracking, depending on the carbon and hydrogen contents and residual stress levels, below about 400°C (preheating and post heating are generally recommended)
- High tensile strength and hardeness Good toughness, particularly for low carbon grades
4 Selecting Shielding Gases for Welding of Stainless Steels (1)
4.1 Influence of the Shielding Gas on: GTAW, PAW, GMAW, FCAW and LBW
The choice of shielding gas has a significant influence on the following factors:
- Shielding Efficiency (Controlled shielding gas atmosphere)
- Metallurgy, Mechanical Properties (Loss of alloying elements, pickup of atmospheric gases)
- Corrosion Resistance (Loss of alloying elements, pickup of atmospheric gases, surface oxidation)
- Weld Geometry (Bead and penetration profiles)
- Surface Appearance (Oxidation, spatters)
- Arc Stability and Ignition
- Metal Transfer (if any)
- Environment (Emission of fumes and gases)
The interaction between the welding process and the shielding gas has been described in greater detail in § 2.
4.2 Selection of Welding Gas
Welding Process | Shielding Gas - Plasma Gas | Backing Gas |
---|---|---|
GTAW | Ar | |
Ar + H2 (up to 20 %) – (1) | Ar | |
Ar + He (up to 70 %) | N2 (2) | |
Ar + He + H2 (1) | N2 + 10 % H2 (1) | |
Ar + N2 (2) | ||
PAW | Idem GTAW | Idem GTAW |
GMAW | 98 % Ar + 2 % O2 | |
97 % Ar + 3 % CO2 | ||
95 % Ar + 3 % CO2 + 2 % H2 (1) | Idem GTAW | |
83 % Ar + 15 % He + 2 % CO2 | ||
69 % Ar + 30 % He + 1 % O2 | ||
90 % He + 7,5 % Ar + 2,5 % CO2 | ||
FCAW | No | No |
97 % Ar + 3 % CO2 | Idem GTAW | |
80 % Ar + 20 % CO2 | ||
LBW | He | Idem GTAW |
Ar |
Ar: argon; H2: hydrogen; He: helium; N2: nitrogen; CO2: carbon dioxide
(1) Hydrogen–containing mixtures must not be used for welding ferritic, martensitic or duplex stainless steels
(2) For welding nitrogen–containing austenitic and duplex stainless steels, nitrogen can be added to the shielding gas
5 Suggested Welding Consumables for Welding Stainless Steels
Base | Material | Welding | Consumables | ||
---|---|---|---|---|---|
EN | 10088 | AISI (1) | EN 1600 | EN 12072 | EN 12073 |
Name | Number | Covered Electrodes (2) | Wires and Rods (3) | Flux Cored Electrodes (4) | |
X5CrNi18-10 | 14301 | 304 | E 19 9 | G 19 9 L | T 19 9 L |
X2CrNi18-9 | 14307 | 304 L | E 19 9 L | G 19 9 L | T 19 9 L |
X2CrNi19-11 | 14306 | 304 L | E 19 9 L | G 19 9 L | T 19 9 L |
X5CrNiTi18-10 | 14541 | 321 | E 19 9 Nb | G 19 9 Nb | T 19 9 Nb |
X5CrNiMo17-12-2 | 14401 | 316 | E 19 12 2 | G 19 12 3 L | T 19 12 3 L |
X2CrNiMo17-12-2 | 14404 | 316 L | E 19 12 3 L | G 19 12 3 L | T 19 12 3 L |
X6CrNiMoTi17-12-2 | 14571 | 316 Ti | E 19 12 3 Nb | G 19 12 3 Nb | T 19 12 3 Nb |
X2CrNiMo18-15-4 | 14438 | 317 L | E 19 13 4 N L | G 19 13 4 L | T 13 13 4 N L |
X10CrNi18-8 | 14310 | 301 | E 19 9 | G 19 9 L | T 19 9 L |
X2CrNiN18-7 | 14318 | 301 L | E 19 9 L | G 19 9 L | T 19 9 L |
X12CrNi23-13 | 14833 | 309 S | E 22 12 | G 22 12 H | T 22 12 H |
X8CrNi25-21 | 14845 | 310 S | E 25 20 | G 25 20 | T 25 20 |
X25CrNiMo18-15-4 | 14438 | 317 L | E 19 13 4 N L | G 19 13 4 L | T 13 13 4 N L |
X2CrTi12 | 14512 | 409 | E 19 9 L | G 19 9 L | T 13 Ti |
X6Cr17 | 14016 | 430 | E 17 or 19 9 L | G 17 or 19 9 L | T 17 or 19 9 L |
X3CrTi17 | 14510 | 430 Ti /439 | E 23 12 L | G 23 12 L | T23 12 L |
X2CrMoTi18-2 | 14521 | 444 | E 19 12 3 L | G 19 12 3 L | T 19 12 3 L |
X2CrTiNb18 | 14509 | 441 | E 23 12 L | G 23 12 L | T 23 12 L |
X6CrMo17-1 | 14113 | 434 | E 19 12 3 L | G 19 12 3 L | T 19 12 3 Nb |
X2CrNiN23-4 | 14362 | – | E 25 7 2 N L | G 25 7 2 L | T 22 9 3 N L |
X2CrNiMoN22-5-3 | 14462 | – | E 25 7 2 N L | G 25 7 2 L | T 22 9 3 N L |
X12Cr13 | 14006 | 410 | E 13 or 19 9 L | G 13 or 19 9 L | T 13 or 19 9 L |
X20Cr13 | 14021 | – | E 13 or 19 9 L | G 13 or 19 9 L | T 13 or 19 9 L |
X30Cr13 | 14028 | 420 | E 13 or 19 9 L | G 13 or 19 9 L | T13 or 19 9 L |
(1) AISI: American Iron and Steel Institute
(2) Covered electrodes for manual metal arc welding of stainless and heat resisting steels. There are two basic flux coverings: basic (B) or lime (direct current) and rutile ( R ) or titania (direct or alternating current)
(3) Wire electrodes, wires and rods for arc welding of stainless and heat-resisting steels: G for G.M.A.W., W for G.T.A.W., P for P.A.W. or S for S.A.W.
(4) Tubular cored electrodes for metal arc welding with or without a gas shield of stainless and heat resisting steels
6 Joint Preparation in Arc Welding
The principal basic types of joints used in arc welding are the butt, lap, corner, edge and T configurations. Selection of the proper design for a particular application will depend primarily on the following factors:
- The mechanical properties desired in the weld
- The type of grade being welded
- The size, shape and appearance of the assembly to be welded
- The cost of preparing the joint and making the weld
No matter what type of joint is used, proper cleaning of the workpieces prior to welding is essential if welds of good appearance and mechanical properties are to be obtained. On small assemblies, manual cleaning with a stainless steel wire brush, stainless steel wool or a chemical solvent is usually sufficient. For large assemblies or for cleaning on a production basis, vapour degreasing or tank cleaning may be more economical. In any case, it is necessary to completely remove all oxide, oil, grease, dirt and other foreign matter from the workpiece surfaces.
6.1 GTAW and PAW
The square-edge butt joint is the easiest to prepare and can be welded with or without filler metal depending on the thickness of the two pieces being welded. Part pos- itioning for a square-edge butt joint should always be tight enough to assure 100% penetration. When welding light gauge material without adding filler metal, extreme care should be taken to avoid lack of penetration or burn through.
The flange type butt joint should be used in place of the square edge butt joint where some reinforcement is desired. This joint is practical only on relatively thin material (1.5 to 2.0 mm).
The lap joint has the advantage of entirely eliminating the need for edge preparation. The only requirement for making a good lap weld is that the sheets be in close con- tact along the entire length of the joint to be welded.
Corner joints are frequently used in the fabrication of pans, boxes and all types of containers. According to the thickness of the base metal, filler metal may or may not be required to provide adequate reinforcement on all corner joints. Make sure that the parts are in good contact along the entire length of the seam.
All T joints require the addition of filler metal to provide the necessary build up. When 100 per cent penetration is required, be sure that the intensity of the welding current is adequate for the thickness of the base material.
Edge joints are used solely on light gauge material and require no filler metal addition. The preparation is simple but this configuration should not be used where direct tensile loads are to be applied to the finished joint, since this type of joint may fail at the root under relatively low stresses.
6.2 GMAW
For GMAW welds, the root opening as well as the V angles can frequently be reduced from those normally employed in SMAW. The amount of weld metal per unit length can thus be reduced up to 30% by providing designs which require less filler metal. When designing GMAW welds for narrow grooves, it is often necessary to employ a high current density (spray transfer).
6.3 FCAW
In butt weld joints, the root openings and V angles can be reduced, often enabling a saving of the order of 40% in the amount of filler metal used in the joint.
The optimum joint design will often be determined by the ease with which slag can be removed in multi-pass welds.
In fillet welding, smaller sizes can be employed for the same strength. The deep penetration capacity of flux cored wire gives the same strength as the larger fillet from a SMAW electrode which has low penetrating power.
By comparison with SMAW electrodes, FCAW wires offer significant cost savings in a variety of ways, such as higher deposition rates, narrower grooves and sometimes two passes before stopping for slag removal.
6.4 SAW
The groove openings are reduced compared to those required by other arc processes. The weld passes are heavier than for SMAW electrodes. For open root configurations, it is often desirable to provide a flux backing held in place by a copper chill bar or by a ceramic bar.
For all processes, bevelling is not required for thicknesses of 3.0 mm and less, but thicker base material should be bevelled to form a “V”, “U” or “J” groove.
7 Finishing Treatments for Welds
The need for surface finishing applies primarily to arc welds. Welds made by resistance welding processes, with the exception of flash butt welding, normally go into service as welded, or after light cleaning.
On completion of an arc welding operation, the weld area and surrounding base material may be contaminated by weld spatter and oxide films, depending upon the joint type, material thickness and welding technique employed.
For maximum corrosion resistance, careful attention should be given to the finishing operation in order to remove all surface contaminants and irregularities that might act as sites for corrosive attack in subsequent service.
In certain applications, where considerations of corrosion resistance, hygiene and aesthetic appeal are paramount, it may be necessary to remove the surplus weld metal and to polish the weld zone to blend with the surrounding base metal.
The normal finishing treatment may be any of the following, used either singly or in combination, depending upon the welding technique and the degree of finish required:
- Mechanical finishing by hammering, brushing, grinding, polishing and buffing;
- Acid pickling followed by passivation treatment and washing.
7.1 Slag, Splatter and Oxide removal
The slag left by welding must be removed by careful hammering, care being taken to avoid denting the adjacent metal surface. Weld spatters are probably one of the most difficult types of contamination to remove, particularly for welds involving highly polished sheets. For this reason, it is usually recommended to protect the sheet surfaces adjacent to the weld with plastic coatings. This precaution confines the area requiring finishing treatment to a minimum.
The removal of oxide films and weld spatter can be achieved by the use of a stainless steel wire brush. If a non-stainless steel brush is used, contamination by iron particles may lead to rust and discoloration during subsequent service.
Another method of removing welding slag and spatter on heavy components is sand blasting. In this process, abrasive particles (silica, alumina, etc.) are projected onto the workpiece with the aid of a high pressure fluid (air or water).
7.2 Grinding
The physical properties of stainless steels require care during grinding to prevent overheating and associated heat discoloration. This phenomenon occurs when the surface temperature exceeds 200°C. In this process, the workpiece surface is abraded with a grinding wheel rotating at high speed, with tangential velocities between 20 and 80 m / sec. The abrasive particles are generally either alumina (corundum) or silicon carbide (carborundum). For rough grinding operations, such as the removal of the excess thickness at a weld 100 – 200 mm diameter cylindrical wheels are employed, with grain sizes of the order of 40 mesh. Depending on the type of binder, the tangential velocity varies from 25 to 60 m / sec. For finish grinding operations (e.g. fine levelling of welds) semi-rigid or flexible abrasive disks are used, with diameters in the range 150 – 250 mm and grain sizes from 80 to 120 mesh, the tangential velocity being between 12 and 15 m / sec.
7.3 Polishing
Polishing is a common surface finishing operation. The normal procedure is to remove the grinding marks using a 180 – 320 mesh abrasive. The tools employed (bobs and mops) must be strictly reserved for stainless steels, in order to avoid all risk of contamination by iron particles. Compared to other materials, the removal of matter requires a great amount of energy for stainless steels. Care must therefore be taken to avoid excessive heating (maximum temperature of the order of 200°C), which can cause slight surface oxidation, hindering the formation of a passive film. The pressure exerted by the disk or belt must be adjusted to the minimum level allowing satisfactory polishing without local overheating.
7.4 Chemical Treatments
7.4.1 Pickling
With certain welding processes, the joint becomes covered with a coloured oxide which it is essential to remove in order to be able to restore passivity. Several techniques can be employed.
➤ Pickling baths for austenitic grades:
52% nitric acid (36° Baumé): 100 l
65% hydrofluoric acid: 20 l
or sodium fluoride: 30 kg
water: 900 l
➤ Pickling baths for ferritic grades:
52% nitric acid (36° Baumé): 100 l
65% hydrofluoric acid: 10 l
or sodium fluoride: 30 kg
water: 900 l
The immersion times at 20°C generally vary between 15 minutes and 3 hours. The bath temperature and immersion time must be carefully controlled to prevent corrosion of the metal. After pickling, the parts must be abundantly rinsed with chlorine-free water.
➤ Pickling pastes and gels:
The use of pastes or gels enables the treatment to be limited to the weld zones. Their compositions vary, but often contain nitric acid. The paste or gel is spread on with a paint brush, and the area is then cleaned with a stainless steel brush. After pickling, the affected area is rinsed with water.
7.4.2 Passivation
After pickling, the metal is unprotected and a new passive layer must be formed to restore its corrosion resistance.
➤ Passivating baths
The parts are immersed in an acid bath with the following approximate composition:
52% nitric acid (36° Baumé): 250 l
water: 750 l
The immersion times at 20°C generally vary between 15 minutes and one hour. After passivation, the parts are carefully rinsed with water.
➤ Passivating pastes and gels
Pastes and gels are used for local passivation of the weld zones. The nitric acid based product is spread on the surfaces to be treated, then totally eliminated by brushing with a stainless steel or nylon brush, followed by rinsing with water.
➤ Decontamination
The different sheet working operations can leave iron-rich particles on the surface, which it is essential to remove. Although their aim is different, the methods used for decontamination are the same as those for passivation.
8 Safe Practices
8.1 Electric shock
In all manual arc welding operations, the principal risk is electric shock, from contact with bare live parts of the welding circuit. The arc voltage is in the range from 10 to 40 volts but, as the voltage required to strike the arc may be higher, power sources have an open circuit voltage up to 80 volts.
Although these voltages appear low compared to the 220 volts of domestic power supplies, it has been shown that only voltages less than 50 volts AC (alternating current) or 120 volts DC (direct current) are unlikely to be dangerous to healthy persons in a dry working environment.
The following guidelines give the principal health and safety precautions required to ensure safe practices and prevent accidents.
- The electrode and workpiece circuits are electrically “live” when the welder is on. Never permit contact between “live” parts of the circuits and bare skin or wet clothing. Appropriate clothing such as gloves, boots and overalls will protect the welder from electric shock.
- Always insulate yourself from the work-piece and ground using dry insulation when welding in damp locations or on metal floors, particularly in positions, such as sitting or lying, where large areas of the body can be in contact with a conducting surface.
- Never dip the electrode holder in water in order to cool it.
- When the welder is used as a power source for mechanised welding, the above guidelines also apply to the automatic electrode, electrode reel, welding head, nozzle or semi-automatic welding gun.
A different kind of electrical hazard can arise from stray welding currents which return to the welding source by paths other than the welding lead. For example, although the return is disconnected, welding is possible when the return current flows through the protective earth conductor of the power source. Stray currents can be comparable in intensity to the welding current when there is poor or faulty insulation of the return lead which may be short circuited by other conductors. When welding on building structures and pipework installations, the welding return lead should be placed as close as possible to the welding area.
8.2 Fumes and Gases
Welding may produce fumes and gases hazardous to health. These fumes and gases can contaminate the atmosphere surrounding the work station. Precautions must be taken to eliminate the risk of such fumes and gases. If not, the fumes and gases must be controlled at their source using a local ventilation and / or exhaust system at the arc to keep them away from the breathing zone. Respiratory equipment should not be used until all other possibilities have been eliminated. In general, protective respiratory equipment is used only as an interim measure but there will be circumstances where in addition to ventilation measures, personal protection may still be necessary.
Do not weld in locations near chlorinated hydrocarbon vapours emanating from degreasing, cleaning or spraying operations. The heat and radiation from the arc can react with solvent vapours to form phosgene, a highly toxic gas, together with other irritating products.
8.3 Arc Radiation
Arc radiation can injure the eyes and burn the skin. The following recommendations should be followed to ensure safe practice and prevent arc burn.
- Use a shield with an appropriate filter and glass screen to protect your eyes from arc radiation and sparks either when welding or when observing open arc welding. Filter lenses should conform to European standards.
- Use suitable clothing to protect your skin from the arc radiation.
- Protect other nearby personnel with suitable screening and warn them not to watch the arc nor expose themselves to the arc radiation, or to hot spatter or metal.
9 Glossary: Terms and Definitions
9.1 Processes
Alternating Current (AC): A kind of electricity which reverses its direction periodically. For 50 cycle current, the current moves first in one direction then in the opposite direction, reversing 50 times per second.
Arc Blow: The deflection of an electric arc from its normal path due to magnetic forces.
Arc Length: The distance from the tip of the electrode to the point where the arc makes contact with the workpiece surface.
Arc Seam Weld: A seam weld made by an arc welding process (e.g. GTAW, PAW, GMAW, SMAW, SAW).
Arc Spot Weld: A spot weld made by an arc welding process (e.g. GTAW, GMAW).
Arc Welding: A group of welding processes in which heat for welding is obtained from an electric arc or arcs, with or without the use of filler metal.
Arcing time factor: The ratio of arcing time to the total time that the power is available.
Automatic Welding: Welding in which the operation is predominantly controlled by a machine.
Direct Current Electrode Negative (DCEN): The arrangement of direct current arc welding leads in which the work piece is the positive pole and the electrode is the negative pole of the welding arc (e.g. GTAW, PAW).
Direct Current Electrode Positive (DCEP): The arrangement of direct current arc welding leads in which the workpiece is the negative pole and the electrode is the positive pole of the welding arc.
Direct Current Reverse Polarity (DCRP) = DCEP
Direct Current Straight Polarity (DCSP) = DCEN
Electron Beam Welding (EBW): A welding process in which heat is generated when a high velocity focussed beam of electrons collides with the workpiece. The welding is normally carried out in a vacuum chamber.
Electroslag Welding (ESW): A welding process based on resistance (RI2) heating of a molten electrically conducting slag. Joints (in a vertical configuration) thicker than 15 mm (with no upper thickness limit) can be welded in one pass, and a simple square–edged joint preparation is used.
Flash Welding (FW): A welding process in which workpieces (bars, tubes) are clamped in a special fixture and brought lightly together. A high current is transmitted through the clamps and the small areas of contact between the workpieces are superheated until molten metal is ejected i.e. flashing.
Flux Cored Arc Welding (FCAW): Metal-arc welding in which a continuous filler metal electrode (tubular electrode) is used. Shielding is provided by a flux contained within the tubular electrode. Additional shielding may or may not be provided by an externally supplied gas mixture.
Gas Metal Arc Welding (GMAW): Metal-arc welding in which a continuous filler metal electrode is used. Shielding of the arc and weld pool is ensured entirely by an externally supplied gas.
Gas Tungsten Arc Welding (GTAW) or TIG Welding: Inert-gas arc welding process using a non-consumable tungsten electrode. A filler metal may or may not be used.
Induction Welding (IW): A welding process in which the heat for welding is obtained from the resistance of the workpieces to the flow of an induced high frequency welding (IHFW) current or induced low frequency welding (ILFW) current, with the application of pressure. The effect of the high or low frequency welding current is to concentrate the welding heat at the desired location.
Inert Gas Welding: Arc Welding in which the arc and weld pool are shielded from the atmosphere by a medium that is wholly (GTAW, PAW processes) or mainly (GMAW, FCAW processes) an inert gas.
Laser Beam Welding (LBW): A welding process in which the heat for welding is obtained from the application of a concentrated coherent light beam focussed on the joint.
Plasma Arc Welding (PAW): Inert-gas arc welding using a non-consumable tungsten electrode.
Projection Welding (PW): A welding process in which small prepared projections on the workpiece surface are melted and collapse when current is supplied through two opposing electrodes.
Resistance Seam Welding (RSEW): A welding process in which the sheets are nipped between two roller electrodes, which travel along the joint to produce a series of spot welds.
Resistance Spot Welding (RSW): A welding process in which the sheets to be welded are clamped between two opposing electrodes, and a high current is passed for a very short time.
Resistance Welding: A welding process in which heat is obtained by electrical resistance heating of the component interfaces, when they are pressed together and a current is passed.
Root run: The first run made in the root of a multipass process.
Semi-automatic Welding: Welding in which the welding operation is partly controlled by a machine, but requires guidance by hand.
Shielded Metal-Arc Welding (SMAW) or Manual Metal-Arc Welding: Metal-arc welding with covered electrodes not exceeding 450 mm in length and applied by an operator. Shielding is obtained by the decomposition of the electrode covering.
Stud Welding (SW): A welding process in which heat is developed with an electric arc struck between the end of a stud and the workpiece component. When the appropriate temperature is reached, the two pieces are brought into intimate contact.
Submerged-Arc Welding (SAW): Metal-arc welding in which a wire electrode or electrodes are used; the arc or arcs are enveloped in a flux, some of which fuses to form a removable slag covering on the weld.
Travel rate: The relative displacement speed between the electrode and the workpiece surface.
Voltage (volts): The voltage as measured at the power source terminals, which determines the arc length. The true arc voltage will always have a lower value at the arc.
Weaving: A technique of depositing weld metal in which the electrode is oscillated.
Welding current (amperes)
9.2 Types of Weld Joint
Angle of bevel: The angle at which the edge of a component is prepared for making a weld.
Bevel: An angular edge preparation.
Backing strip: A piece of material placed at a root and used to control the penetration of a weld.
Butt joint: a joint between the ends or edges of two abutting members aligned approximately in the same plane (i.e. making an angle to one another close to 180°).
Butt weld: A weld in which the weld metal is deposited within the edge of a butt joint.
Chamfer: Another term for bevel.
Closed joint: A joint in which the surfaces to be joined (edges of two parts) are in contact while being welded.
Concave fillet weld: A fillet weld in which the weld face is concave.
Corner joint: A joint between the ends or edges of two parts making an angle of more than 30° but less than 135°.
Cruciform joint: A joint in which two flat plates are welded to another flat plate at right angles and in the same axis.
Double V butt weld: A butt weld in which the prepared edges of both components are double bevelled so that in cross-section the fusion zone forms two opposing V’s.
Edge joint: A joint between the edges of two parts making an angle to one another close to 0°.
Edge presentation: Squaring, grooving or bevelling an edge in preparation for welding.
Fillet weld: A weld of approximately triangular cross-section joining two surfaces approximately at right angles to each other in a lap joint, T joint or corner joint.
Flat fillet weld: A fillet weld in which the weld face is flat.
Fusion line: The junction of the weld metal with the un-melted base material.
Gap or root opening: The distance at any cross-section between the edges, ends or surfaces to be joined.
Heat affected zone (HAZ): The portion of the base metal immediately adjacent to the fusion line, which has not been melted, but in which the microstructure has been affected by the heat of welding.
Joint: A junction, that is to be welded, between two or more workpieces of between two or more parts of a single workpiece.
Lap joint: A joint between two overlapping parts making an angle to one another close to 0°.
Open joint: A joint in which the parts to be joined are separated by a specified gap while being welded.
Overlap: Protrusion of weld metal beyond toe, face or root of a weld.
Penetration: The depth to which of the fusion zone extends below the surface of the part or parts being welded.
Root of a joint: The zone where the members were closest before welding.
Single V butt weld: A butt weld in which the prepared edges of both parts are bevelled so that in cross-section the fusion zone forms a V.
Square butt weld: A butt weld in which the abutting edges lie approximately at right angles to the surface of the components to be joined and are parallel to one another.
T (or tee) joint: A joint between the end or edge of one part and the face of another part, the two components making an angle close to 90°.
Tack weld: A light weld used to assist assembly or to maintain alignment of edges during welding.
Throat thickness: The minimum thickness of a weld measured on a line running from the root and passing through a point midway between the toes.
Undercut: A groove melted into the base metal adjacent to the toe of the weld and left unfilled by weld metal.
Y joint: A joint between the end or edge of one part and the face of another, the parts making an angle to one another of more than 10° but less than 70°.
Weld face: The exposed surface of a fusion weld on the side from which the weld has been made.
Weld penetration: The depth of fusion or melting as measured from the original sur- face of the base metal.
Weld reinforcement: The built-up weld metal on the face of the weld in excess of the specified weld thickness.
9.3 Welding Consumables
Covered electrode: A filler rod having a covering flux (for SMAW) used in arc welding, consisting of a metal core with a relatively thick covering which provides protection for the molten metal and stabilises the arc.
Filler metal: Metal added during welding (brazing or surfacing).
Filler rod: Filler metal in the form of a rod (e.g. for GTAW).
Filler wire: Filler metal in the form of a coil of wire (e.g. for GMAW and SAW).
Flux: A fusible material used to protect the weld from atmospheric contamination, to stabilise the arc and to perform a metallurgical function (to prevent, dissolve, or facilitate removal of oxides and other undesirable substances).
Flux cored electrode: Filler metal in the form of a small tube with flux in the core. The core provides deoxidisers and slag-forming materials and may also provide shielding gases (some flux cored electrodes are self-shielding).